Ptychography is a very powerful coherent diffractive imaging (CDI) technique that has recently gained remarkable momentum in optical microscopy. In ptychography, a complex-valued object is scanned in a step-wise fashion through a localized coherent beam. In each step, the far-field diffraction intensity is measured. Critically, the illumination spot in each step overlaps substantially with neighboring spot. The set of diffraction patterns is used for reconstructing the complex object.
Here we address two major limitations in ptychography: i) the need for scanning, which poses a major limitation of the data acquisition time, and ii) diffraction-limit spatial resolution. First, we propose and experimentally demonstrate single-shot ptychography, where all diffraction patterns from array of partially overlapping illuminating spots are recorded in a single exposure. Whereas scanning limits the acquisition time of ptychgraphic microscopes to the range of ~0.1secs, single-shot ptychography allows for ultrafast ptychographic microscopy. Second, we demonstrate sub-wavelength ptychography, utilizing the fact that the sought information (the complex field of the object) can be represented compactly (sparsely) in real space or in a suitable mathematical basis. As an example, we present ~3.5 times resolution enhancement (beyond the Abbe resolution limit) of a biological specimen.
For single-shot ptychography, a coherent monochromatic plane wave illuminates a square array of N×N pinholes positioned at the input face of a 4f system (Fig. 1a). The object and CCD are located at distance d≠0 before the Fourier plane and at the output plane of the 4f system, respectively. Lens L2 transfers the field after the object to k-space domain at the CCD plane. The detected intensity pattern consists of clearly distinguished N2 diffraction patterns, where each pattern can be associated with a beam illuminating the object at a specific given spot. Figure 1 presents experimental single-shot ptychography. The spatially coherent light illuminates an N2=49 square array pinholes. The measured intensity pattern is displayed in Fig. 1c. Figures 1d and 1e show our single-shot ptychographically reconstructed amplitude and phase,
respectively, using ePIE algorithm [1].
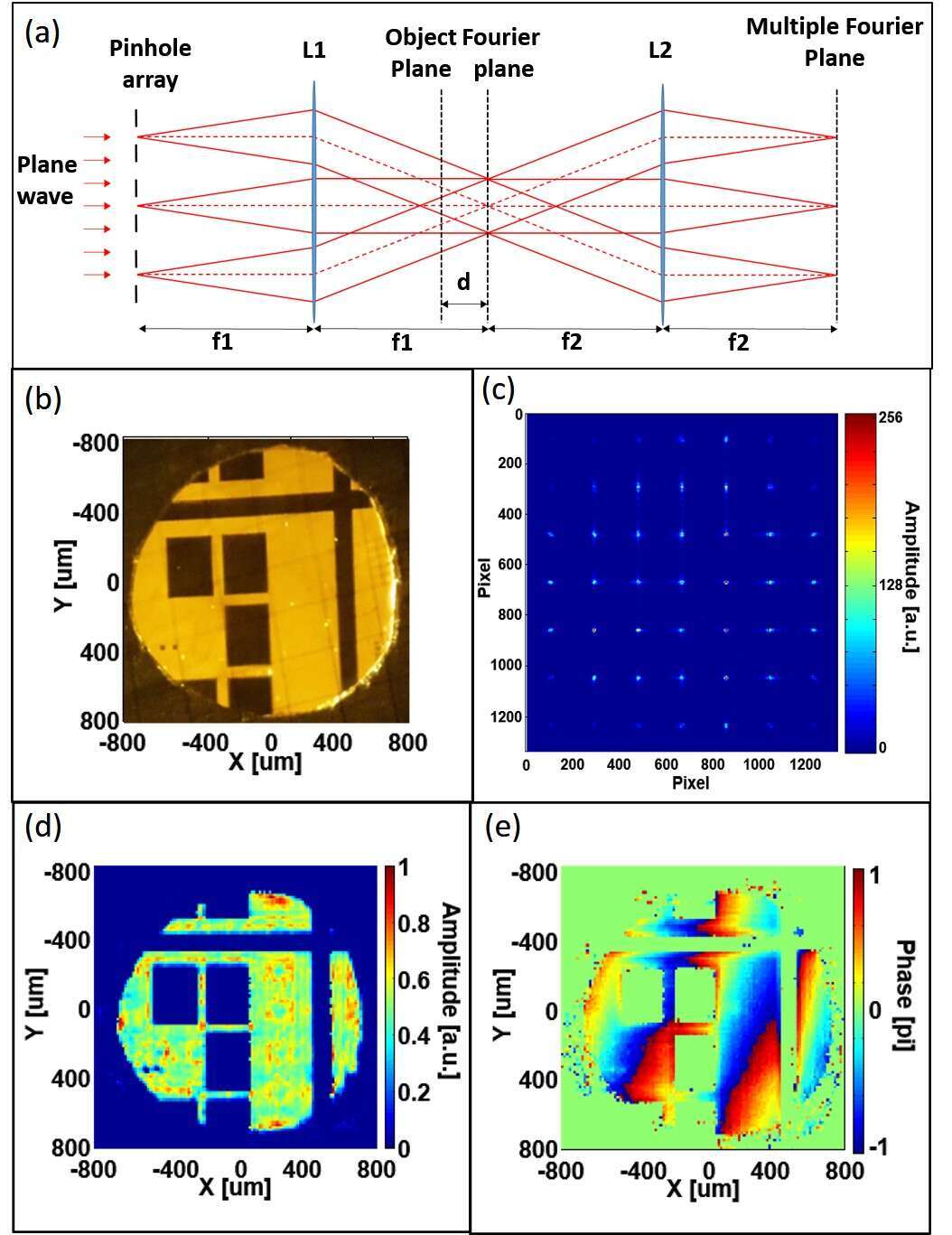
Next, we move to subwavelength ptychography by employing sparsity, i.e. prior information that the image can be represented compactly in a known physical or mathematical basis. Previously, we demonstrated experimentally sparsity-based sub-wavelength CDI of relatively simple objects [2]. Implementation of the same approach in ptychography yields sub-wavelength resolution of “real world” objects because the number of measurements in ptychography is much larger than the number of degrees of freedom of the sought image. Figure 2 presents a numerical example. Figure 2b shows the diffraction limited ptychographic reconstruction while the sparsity-based reconstruction, that searches for the best estimate of the object that is consistent with the set of measurements and is sparse in known mathematical basis, is shown in Fig. 2c. Figure 2d shows a lineout of the original image, its diffraction limited image and the sparsity-based reconstruction. Clearly, the sparsity-based reconstruction yields subwavelength resolution.
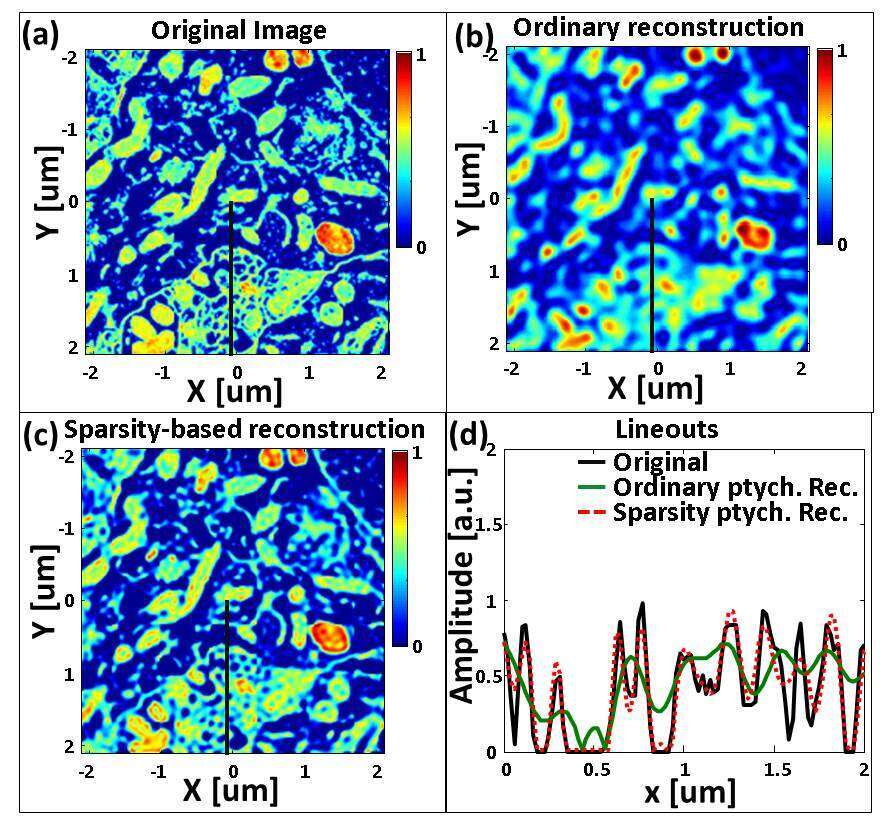
References
[1] Maiden et al., Ultramicros. 109, 1256-1262 (2009).
[2] Szameit et al., Nat. Mat. 11, 455 (2012).
[4] Sidorenko et al., Nat. Commun. 6, 8209 (2015)