On the molecular level, carbon nanotubes (CNTs) exhibit excellent mechanical strength, thermal and electrical conductivity properties, combined with low density. This unique-feature combination makes CNTs ideal candidates for processing multifunctional macroscopic fibers. However, translating the unique characteristics of a single molecule into macroscopic material is challenging.1,2
The most significant limitation that prevents wide-scale use of CNTs in applications is the difficulty of dispersing CNTs to form thermodynamically stable solution, free of aggregates or bundles. Recently it was discovered that CNTs spontaneously dissolve in chlorosulfonic acid (CSA), forming a molecular solution, namely, the nanotubes are dispersed as individual molecules, and at higher concentrations form a lyotropic liquid crystalline nematic phase.3 Alignment of CNTs in a solution is essential to achieve the best properties in a CNT-based macroscopic material.4
CNT parameters, such as length, diameter, and degree of purity, dictate CNTs solubility in acid, phase transition concentration from isotropic to biphasic, where isotropic and nematic phases coexist, CNTs arrangement in the biphasic, and the fully nematic phase. Therefore, understanding the influence of CNT parameters on the CNT/CSA system phase behavior, and specifically, the formation of liquid crystalline phase, is necessary for designing a successful CNT fluid-phase process, while preserving CNTs’ unique molecular properties.
In this study we characterize CNT/CSA solutions with different CNT parameters, such as length and diameter, by a combination of polarized light microscopy and cryogenic electron microscopy. Results demonstrate that solutions with higher aspect ratio CNTs, phase separate at much lower concentrations (Fig. 1), in agreement with Flory`s rigid-rod theory.5 In addition, there is a difference in nematic phase morphology as a function of aspect ratio, by means of CNTs arrangement within the ordered phase.
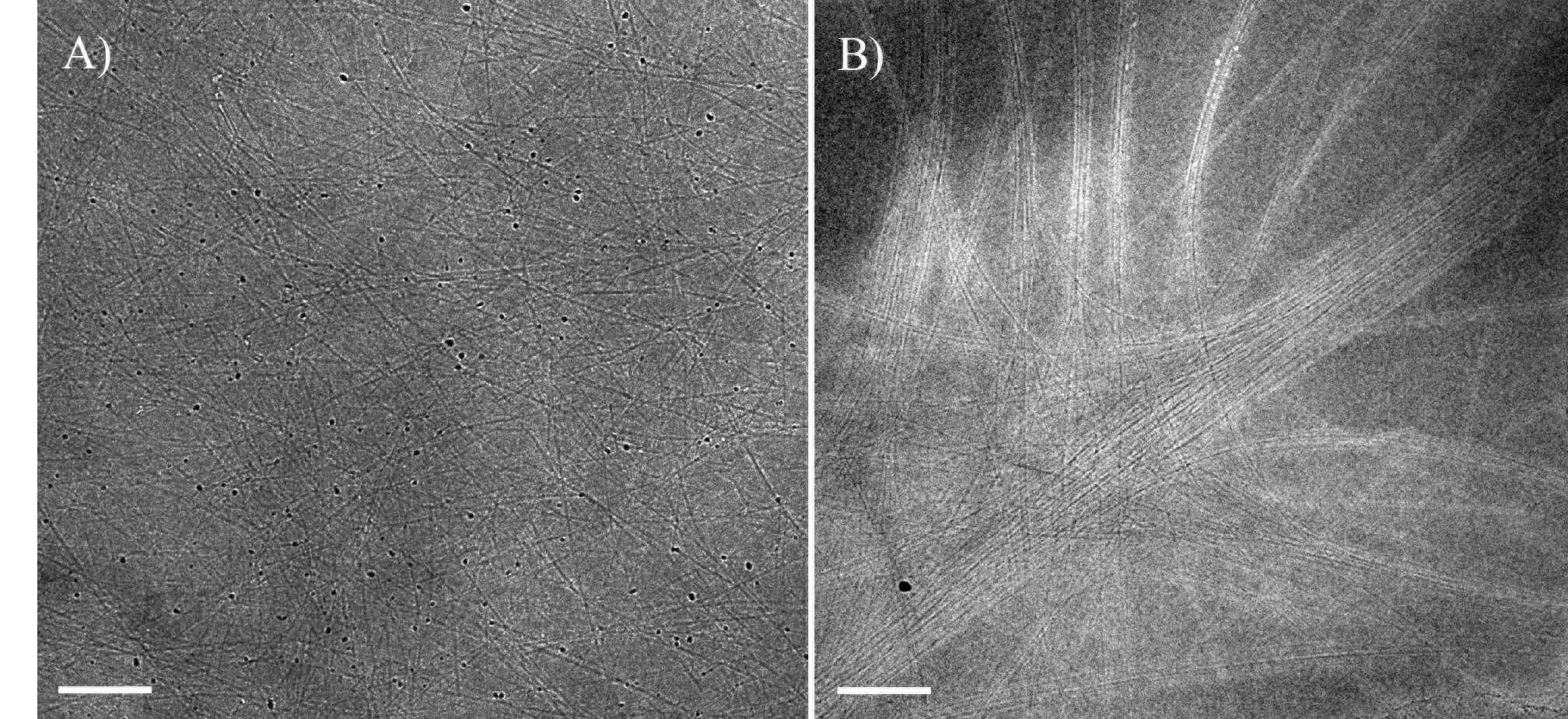
Figure 1: Cryo-TEM images of (A) short HiPco SWNTs at 250 ppm in isotropic solution (phase separation occurs at 1400 ppm). (B) long Meijo SWNTs at 100 ppm in bi-phasic solution (phase separation occurs at 100 ppm). Bars correspond to 100 nm.
Bibliography
(1) Behabtu, N.; Young, C. C.; Tsentalovich, D. E.; Kleinerman, O.; Wang, X.; Ma, A. W.; Bengio, E. A.; ter Waarbeek, R. F.; de Jong, J. J.; Hoogerwerf, R. E.; et al. Strong, Light, Multifunctional Fibers of Carbon Nanotubes with Ultrahigh Conductivity. Science 2013, 339, 182–186.
(2) Kleinerman, O.; Parra-Vasquez, A. N. G.; Green, M. J.; Behabtu, N.; Schmidt, J.; Kesselman, E.; Young, C. C.; Cohen, Y.; Pasquali, M.; Talmon, Y. Cryogenic-Temperature Electron Microscopy Direct Imaging of Carbon Nanotubes and Graphene Solutions in Superacids. J. Microsc. 2015, 259, 16–25.
(3) Davis, V. A.; Parra-Vasquez, A. N. G.; Green, M. J.; Rai, P. K.; Behabtu, N.; Prieto, V.; Booker, R. D.; Schmidt, J.; Kesselman, E.; Zhou, W. True Solutions of Single-Walled Carbon Nanotubes for Assembly into Macroscopic Materials. Nature Nanotechnol. 2009, 4, 830–834.
(4) Zakri, C. Carbon Nanotubes and Liquid Crystalline Phases. Liq. Cryst. Today 2007, 16, 1–11.
(5) Flory, P. J. Phase Equilibria in Solutions of Rod-Like Particles. Proc. R. Soc. A Math. Phys. Eng. Sci. 1956, 234, 73–89.