The purpose of this work is to obtain experimental data about shock waves and gas-dynamic parameters near the extended breakdown plasma in the supersonic air flow; to study interaction between the thermal wake and the shock-wave from the flowed body.
The supersonic stationary air flow corresponding to the Mach number Маха М = 1.36 was organized in the wind tunnel; the profiled nozzle with the cut diameter dс = 50 mm was applied. The experiments were carried out without Eifel chamber, the pressure in the settling chamber was Рo= 0.3 MPa (design flow mode, flow density 1.8 kg/m3) and 0.5 MPa (off-design flow mode, flow density in the focusing area 2.8 kg/m3). For the flow structure visualization, a system of shadow and Schlieren measurements was utilized.
The optical breakdown was formed at the focusing (f = 63 mm) of the impulse-periodical radiation of a СО2 laser with the mechanical Q-switching. The average generation power was up to 3.5 kW, pulse repetition rate from 54 kHz to 150 kHz, peak power – up to 200 kW. The laser radiation was input perpendicularly to the supersonic flow velocity vector. The diameter of the radiation spot in the lens focus was about 100 – 150 mm, which provided the specific power in the optimum operation mode of the laser of about (7 – 15)*108 W/cm2.
Fig. 1 shows the flow pattern obtained by the Schlieren method. The photos show the shock waves from the laser sparkle and low-density areas which result from the ansorption of the CO2 laser energy by the breakdown plasma. Ahead of the energy-outflow area, the head shock wave forms.
With the shot exposition time (150 ns), it is possible to see the dynamics of propagation of individual low-density areas in the supersonic flow. The wake structure consists of localized almost-spherical low-density disturbance areas; their size slightly varies downstream. The distance between the cavities decreases as the pulse repetition frequency rises.
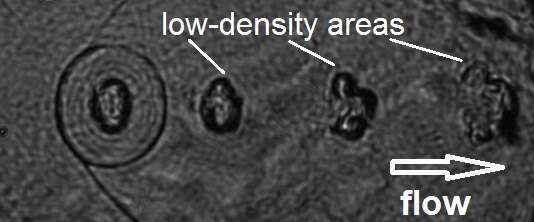
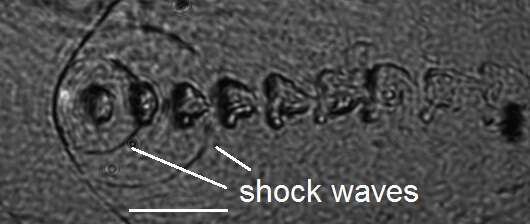
Fig. 1. Schlieren images of the flow: (а) the pulse-periodical radiation frequency - 60kHz, (b) -60kHz.
Fig. 2a shows the flow with the laser plasma moving around the model (the flow pattern averaged by 30 pulses), Fig. 2b presents a shot of the same, the pulse repetition frequency is 150 kHz. In the supersonic flow, along with the shock waves generated by the model, there is a head shock wave forming ahead of the energy-release area; it is an envelope for the shock waves generated by periodical optical discharges as the explosion center is shifted downstream with the flow speed.
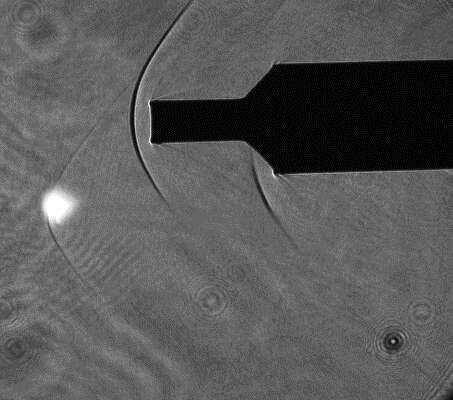
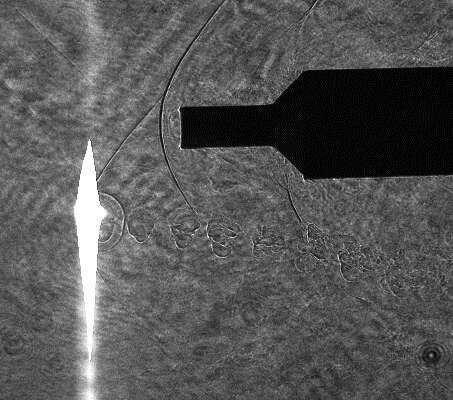
Fig. 2. Schlieren images of the flow. (а) – shot exposure time 200 microseconds, (b) shot exposure time 150 ns.
Both shock waves from the model registered as the energy release is missing in the studied area, are not observed below the thermal wake if the laser radiation energy is supplied into the flow. It means that high density gradients really undergo changes (reduce) and probably quite dramatic weakening of the shock waves takes place as they are interacting with the thermal wake.
- Georgievskij P.Yu., Levin V.А. Supersonic flow around the bodies at the presence of external sources of heat release // Letters to the Journal of Applied Physics. 1988. Vol. 14. Issue 8. Pp. 684-687.
- Tret’jakov P.К., Garanin А.F., Grachevв N., Krajnev V.L., Ponomarenko А.G., Ivanchenko А.I., Yakovlev V.I. Control of the supersonic flow around bodies with the powerful optical discharge / / DAN. 1996. Vol. 351, No. 3, pp. 339-340.
- Malov А.N., Orishich А.М., Bobarykina Т. А., Chirkashenko V.F. Absorption of СО2-laser radiation in the optical discharge plasma in a supersonic air flow // XV International Conf. on Methods of Aerodynamical Research. Novosibirsk, 2010г. Part 2. - p.157.