Introduction
Prediction of the radiative portion of the heatflux expected by a spacecraft entering an atmosphere, still poses a challenge even to the state of the art numerical models. On ground testing facilities these atmospheric entry flows can be simulated using a superorbital expansion tunnel, where the test gas used in the facility is a representative atmospheric mixture of the celestial body of interest. Entry into the atmosphere of Titan, the moon Saturn, was studied using the X2 superorbital expansion tunnel. For Titan atmospheric entry conditions, the radiative heat transfer is expected to dominate the shock layer, at what is considered to be a relatively low shock speed above 6km/s. This is a result of the carbon content in Titan’s atmosphere, leading to the formation of CN, a strong radiator. To further our understanding of superorbital flows, the experiments use newly developed radiation gauges to measure the radiative heatflux, and emission spectroscopy to provide information about the radiating species in the shock layer.
Experimental Conditions
Experiments were conducted using a Titan 6.5km/s atmospheric entry condition. It simulates peak radiative heating for Titan entry at the stagnation point, using a test flow with an equivalent flight velocity of 6.8km/s, Mach 11, 150μs test time. Impulse facilities test time are short and typically between 50-150μs. Fast response and recording equipment are sufficient to gather the data during the experiment. The test gas used was a mixture of 95% Nitrogen to 5% Methane (by volume).
Experimental Arrangement
Two models were used in the experimental campaign: a cylindrical and a hemispherical model. The purpose of the two models was to confirm the hypothesis that the same amount or radiation will be measured if the appropriate scaling is applied to keep the same shock standoff.
A 75mm cylindrical model, with 25mm diameter was instrumented with two radiation gauges along the stagnation streamline. The cylindrical model was setup in the X2 expansion tunnel, and radiative heatflux measurements were made in parallel with emission spectroscopy. The optical arrangement for the emission spectroscopy can be seen in Figure 1. It was designed to image the region between the shock and the body along the stagnation streamline over the wavelength range 320-440nm. The area of interest was set to view the CN violet bands (spectral lines around 358nm, 384nm and 418nm) that are the main source of radiation for Titan atmospheric entry.
For the comparative radiative heatflux measurements, a hemispherical model with 75mm diameter was used, instrumented with a single radiation gauge at the stagnation point. The hemispherical model was scaled from the cylindrical model, using empirical correlations of the shock standoff. The calculations made indicated that scaling the hemispherical radius by 3 will result in the same shock standoff as the cylindrical model. Hence the 25mm diameter cylinder was scaled to a 75mm diameter hemisphere to test the hypothesis, that the same shock standoff will results in a comparative level of radiative heat transfer.
Radiation Gauge
A new radiation gauge was developed, using a multi-walled Carbon Nanotube (CNT) thin film sensing element. The new gauge, CNT-RAD, was coated on a macor substrate with the CNT sensing element at the Indian Institute of Science in Bangalore, using a one-step pyrolysis process [2]. The gauge was wired using silver tabs that were applied along the sides as seen in Figure 2. After calibration the gauge was placed in brass housing, and set behind a viewing window. This configuration was proved to allow measurements of the radiative heatflux, and is independent of the convective heatflux. The absorption of the CNT sensing element was measured, and the correction was applied to the results.
Results
The measured heatflux using the hemispherical model (denoted h), and a cylindrical model (denoted c), is shown in Figure 3. The test time in these experiments was between 2125-2200μs, where steady heatflux is evident. As the hemispherical model was scaled from the cylindrical model in order to have the same shock standoff, so that the radiating gas in the shock layer for both the cylinder and the hemisphere will be the same, agreement was expected between the radiative heatflux measurements. Referring to Figure 3, it is evident that an agreement between the results was observed. The measured heatflux averaged over 50μs of test time was found to be: 0.46±0.08 MW/m2 and 0.45±0.06MW/m2 for the cylindrical and hemispherical models respectively.
Conclusions
The radiative heatflux for a Titan 6.5 km/s entry condition was successfully measured by newly developed CNT-RAD radiation gauges. Radiative heatflux measurements were made using cylindrical and hemispherical models, confirming that scaling the shock standoff has successfully resulted in comparable radiative heatflux measurements. The spectral distribution and radiative intensity was also measured for the cylindrical model along the stagnation streamline. The results show that CN violet bands are dominating the spectra, and can be analysed to allow a temperature analysis to further characterise the flow.
![Figure 1: Optical arrangement for emission spectroscopy (first published in [1])](http://events.eventact.com/Ortra/16496/ISSW_1.png)
Figure 1: Emission Spectroscopy[1]
![Figure 2: CNT-RAD radiation gauges (i) The polished face of the macor with the CNT coating (ii) The surface of the gauge and the silver connection tabs (iii) Insulated wires soldered to the silver tabs (iv) The assembled gauge in the brass housing behind a window (first published in [2])](http://events.eventact.com/Ortra/16496/ISSW2.png)
Figure 2: Radiation Gauges[2]
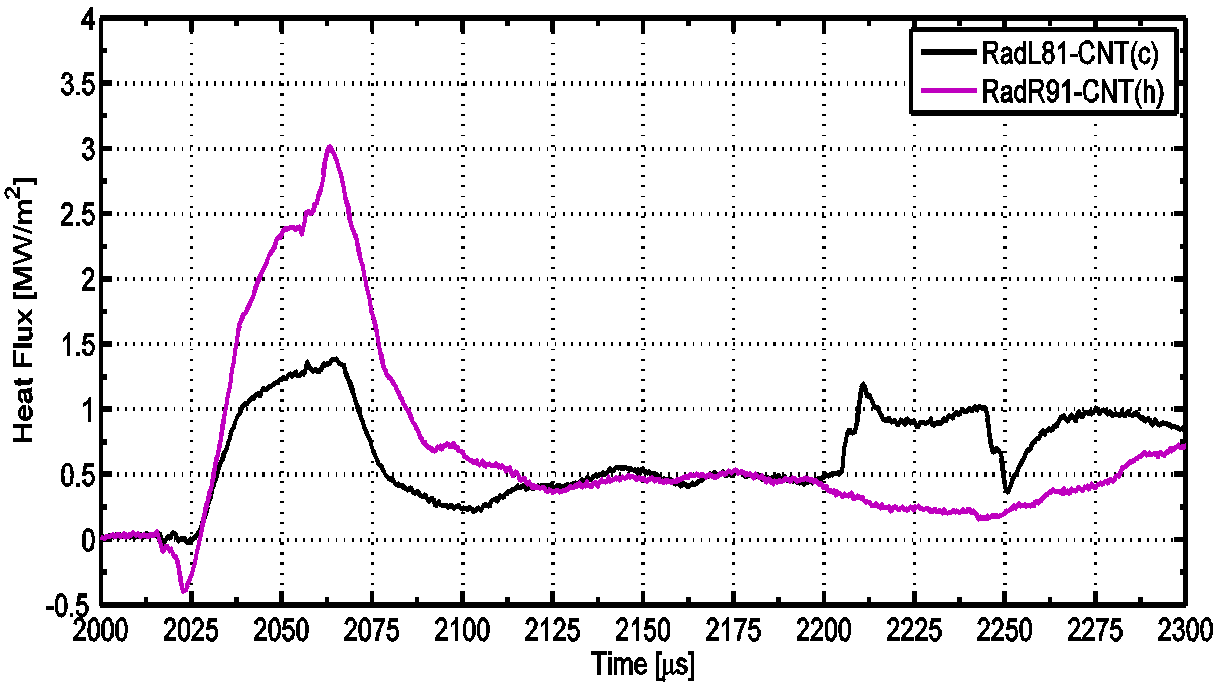
Figure 3: Radiative Heatflux
References
[1] H. Porat, R.G. Morgan, T.J. McIntyre, ‘Study of Radiative Heat Transfer in Titan Atmospheric Entry’, 28th International Congress of the Aeronautical Sciences, July 2012, Brisbane
[2] H. Porat, B. Allsop, R.G. Morgan, S. Srinath, K.P.J. Reddy and T.J. McIntyre, ‘Amplifier Design for New CNT Radiation Gauges in Expansion Tunnels’, 19th Australasian Fluid Mechanics Conference, paper accepted, December 2014, Melbourne