Wave experiments have been carried out in a shock tube to study water condensation (Fransen et al. 2013). Water condensation plays an important role in a variety of industrial processes and is relevant for both cloud formation and climate change models. Water condensation experiments are usually performed at (sub)atmospheric pressures, which are less relevant for technological applications. We have studied water condensation in several gases at 1.0~MPa. To determine the exact amount of water in the gas-vapor mixture, we implement Karl Fisher Titration (KFT) and Chilled Mirror Hygrometry (CMH) techniques (Fig. 1). The KFT and CMH devices are connected to the High Pressure Section (HPS) of the shock tube by 3-way valve V1. This allows us to sample both upstream and downstream of the HPS. This is of importance because of possible water adsorption at the HPS walls. The gas volume going to the KFT device is measured by a rotameter (RM) and both temperature (T) and pressure (P) are measured. Combining both techniques significantly reduces the measurement uncertainty.
Fig. 1 The shock tube with KFT and CMH.
The shock tube is specifically designed to produce a nucleation pulse so that we can measure the nucleation rate. The nucleation rate describes the number of water droplets that are formed per unit of time and volume. The nucleation pulse creates a state of high supersaturation (ratio of partial water pressure and saturation vapor pressure) in which the nucleation of droplets takes place. Because the nucleation is confined to a small time interval all droplets have the same size. Droplet growth takes place in the period of constant pressure and supersaturation after the pulse so that nucleation and growth of droplets are effectively decoupled. The droplet growth is studied by combining two different optical techniques based on light scattering (Fransen et al. 2013). Once the droplet growth curve (droplet radius versus time) is determined, the droplet number density follows from the measured extinction. The nucleation rate is finally obtained as the ratio of the droplet number density and the nucleation pulse duration.
Figure 2 shows new experimental nucleation rates for water in helium at 219 K and 1.0 MPa. They are compared with data by Holten et al. (2005) for helium at 224 K and 97 kPa (obtained with the same shock tube) and data by Wölk and Strey (2001) for argon at 219 K and 50 kPa (obtained with an expansion chamber). The type of carrier gas is not expected to influence the results because only noble gases are used. Comparing our data with those by Wölk and Strey at exactly the same temperature (219 K which is well below the freezing point of water) suggests that the nucleation rate is strongly depending on pressure. This effect is not visible when comparing our data with those by Holten et al., but we note that their experiments were carried out at a higher temperature (224 K). It is well known that the Classical Nucleation Theory (CNT) is not able to predict the correct nucleation rates under these circumstances (Fig. 2). Interestingly, the shock tube experiments significantly extend the range of data to higher values for supersaturation and nucleation rate than previously reported. This offers the possibility of validating new and improved nucleation theories over a wider range of values.
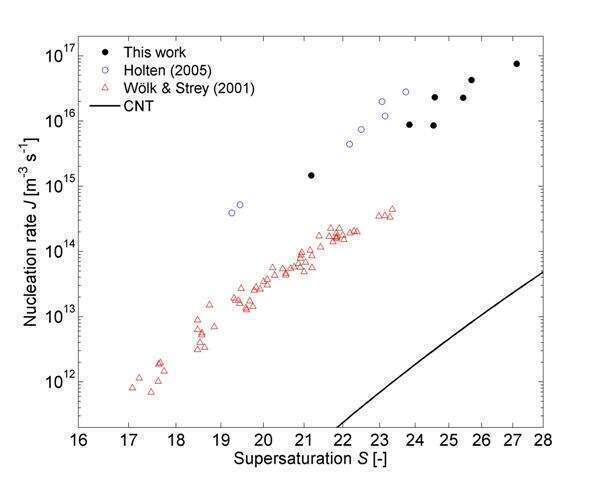
Fig. 2 The experimental nucleation rate for water in helium at 219 K and 1.0 MPa compared with water in helium at 224 K and 97 kPa by Holten et al. (2005) and water in argon at 219 K and 50 kPa by Wölk and Strey (2001). CNT predictions for our conditions are also shown.
REFERENCES
(1) Fransen et al. 2013, Fransen MALJ, Sachteleben E, Hrubý J, Smeulders DMJ (2013) Wave-induced droplet growth measurement by laser light. 29th International Symposium on Shock Waves, Madison (WI), United States, pp. 71
(2) Wölk and Strey 2001, Wölk J, Strey, R (2001) Homogeneous nucleation of H2O and D2O in comparison: the isotope effect. J. Phys. Chem. B 105:11683--11701
(3) Holten et al. 2005, Holten V, Labetski DG, van Dongen MEH (2005) Homogeneous nucleation of water between 200 and 240 K: New wave tube data and estimation of Tolman length. J. Chem. Phys. 123:104505