The development of reaction mechanisms describing the combustion chemistry of jet fuels requires experimental data as targets for these kinetics models. To this end, species time-histories were measured using laser absorption behind reflected shock waves during the pyrolysis and oxidation of a variety of kerosenic jet fuels. Species measured include: fuel, ethylene, and other important jet fuel decomposition products including methane, iso-butene, and acetylene. Initial reflected shock conditions cover temperatures from approximately 1000 to 1400K and pressures from 1 to 20 atm.
In this study, measurements of the pyrolysis and oxidation systems of real fuels, rather than of surrogates or solvent surrogates were acquired as these are needed to provide a direct link to actual fuel behavior. This data, therefore, provides development and validation targets for hybrid kinetic models that are based on real fuel decomposition products such as proposed by Prof. Hai Wang of Stanford University (Hybrid, experimentally constrained pyrolysis – detailed oxidation (Hybrid ECP-DO) model, H. Wang, AFOSR Contractors Meeting June 3, 2014).
Measurements were made behind reflected shock waves in the helium-driven 5 cm diameter Stanford high-pressure shock tube. Fuel concentration time-histories were measured using laser absorption at 3.39 microns using an IR HeNe laser. Ethylene concentration time-histories were measured using laser absorption at 10.53 microns using a CO2 gas laser. Methane time-histories were measured using laser absorption at 3.4 microns using a DFB diode laser.
Representative species time-histories for fuel (JP-8 POSF 10325) and two major decomposition products, ethylene and methane, are shown in Figs. 1 and 2. Also shown are simulations using the initial Hybrid ECP-DO model approach of Prof. Hai Wang. Excellent agreement with measurement is achieved using this approach.
The strategy for hybrid kinetics model development used here builds on earlier work in our laboratory to study the decomposition of RP fuels. In that study, decomposition yield data for selected kerosene-based fuel surrogates were acquired (M. E. MacDonald, W. Ren, Y. Zhu, D. F. Davidson, R. K. Hanson, Fuel 103 (2013) 1060-1068). Representative data from that study are shown on Fig. 3. The wide variety of ethylene yields recovered for different surrogate components, components that are often considered to be archetypal fuel class surrogates, indicates that only a careful tuning of the surrogate composition based on actual measured jet fuel decomposition products will recover the correct product yields and kinetics behavior during jet fuel pyrolysis. Previously used surrogate-based approaches where only physical-chemical parameters of jet fuel are matched are unlikely to accurately duplicate the chemical behavior of the fuel.
The hybrid model approach, supported here with species time-history measurements of real jet fuels, greatly increases the likelihood of developing compact models for jet fuel chemistry.
This work was supported by Air Force Office of Scientific Research under the Basic Research Initiative Program Grant Number FA9550-12-1-0472 with Dr. Chiping Li as Program Manager.
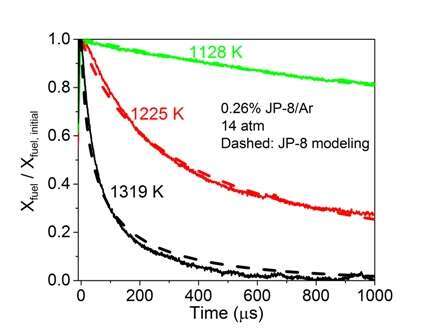
Figure 1. A comparison of the measured and modeled fuel time-histories during jet fuel pyrolysis for several temperatures. Initial reflected shock conditions are 1128 K, 1225 K, and 1319 K, at 14 atm with 0.26% jet fuel in argon.
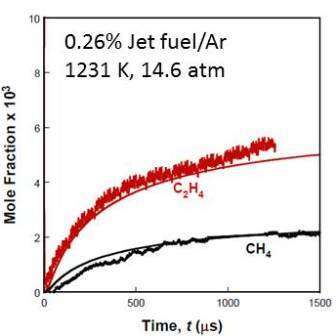
Figure 2. A comparison of the measured and modeled C2H4 and CH4 time-histories during jet fuel pyrolysis. Initial reflected shock conditions 1231K, 14.6 atm, 0.26% jet fuel in argon.
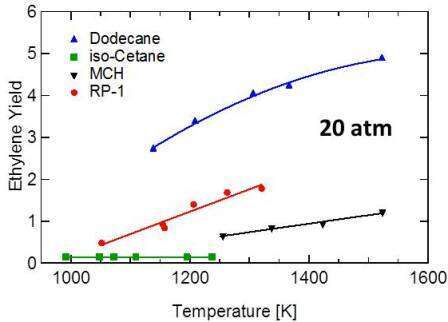
Figure 3. Ethylene yields for selected jet fuel surrogate components. Wide variations in ethylene yield are apparent and these yields are not related to the physical-chemical properties of the surrogate component.