1. Introduction
Shock wave-boundary layer Interaction (SWBLI) causes many serious problems to supersonic transport, such as unsteadiness of flow which leads to a decrease of engine performance, wing lift capacity and control surfaces effectiveness, as well as heat transfer and pressure loads which reduce the endurance of aircraft structures. To realize the future supersonic transport, one of the most important problems is to minimize the negative impact of SWBLI on it.
There are many studies about control of flow in the SWBLI region. Raghunathan[1] investigated methods of passive flow control. Bahi et al,[2] used cavity to connect the high pressure region behind the shock wave and the low pressure region in front of the shock wave. Kontis et al,[3] suggested a passive flow control method using strakes to delay separation by transferring momentum from free stream. However, these methods can not control flow actively and increase of skin friction drag because of added structures.
One of the methods of active flow without attaching structure is energy deposition. From previous research[4], laser energy deposition was successfully applied to suppress the separation of boundary layer in SWBLI by using the interaction between boundary layer and low-density-bubbles created by high repetitive laser pulses. In this study, low-frequency unsteadiness of shock wave in SWBLI is investigated by focusing on the oscillation of shock foot position in interaction region. Understanding the mechanism of phenomenon, the objective of this study is to investigate the application of energy deposition in order to control and mitigate this oscillation.
2. Experimental setup
Figure 1 is the schematic of the experimental setup. This study is carried out by supersonic wind tunnel, the flow Mach number is 1.92. The test section is 80 mm × 80 mm square cross section. The running time of the wind tunnel is 5s. Upper stream side is open, and downstream side is connected to the vacuum chamber. The source of energy deposition was Nd:YVO4 laser (Edgewave, HD401-E, wavelength: 1064 nm; 5.5 mJ / pulse; repetition frequency: up to 100 kHz; average power: up to 400 W). To visualize the flow field, a Schlieren system was deployed using a high speed framing camera (HS-106E, NAC Co., 360x410 pixels, 1.25x106 fps in 120 frames) and Z-shaped mirror and lens system. To evaluate the aerodynamic forces acted on testing model, an internal force balance was used for lift, drag and pitching moment measurement in small cross section wind tunnel. The hemisphere nose-cylinder-flare model (Fig. 2) was continued investigating in this study.
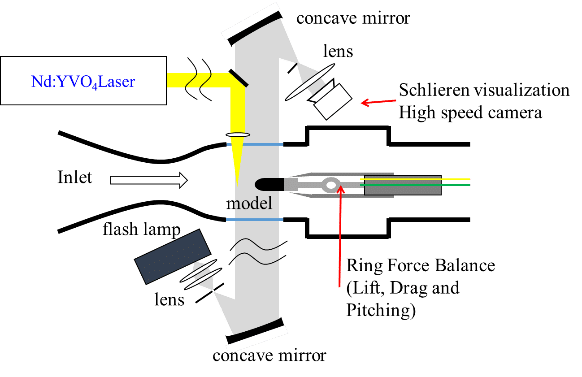
Fig.1 Experimental setup, in-draft supersonic wind tunnel, cross-sectional top view
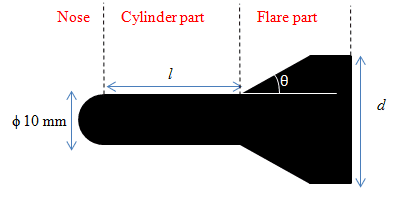
Fig.2 Experimental model
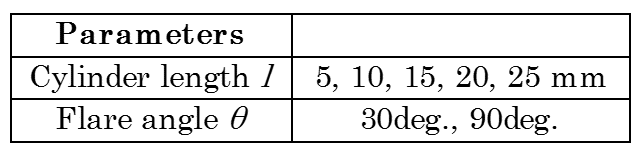
Table.1 Model configuration
3. Results
Figures 3-6 shows the Schlieren images without energy deposition and with energy deposition with laser pulse repetition frequency of 60 kHz. The SWBLI can be seen in front of flare. In the case of =15mm and =30 deg. model, without energy deposition(Fig.3 (a)) and with energy deposition(Fig.3 (b)), it is not observed that large scale motions of shock foot. In the case of =25mm and =30 deg. model, without using energy deposition(Fig.4 (a)), there is not only the separation of boundary layer by interacting with the shock wave but also the repeated motion of the shock foot during this interaction. A shock wave was formed further upstream, and then moved downstream, as well as the separation became larger when the shock foot moved from cylinder part to flare part. With 60 kHz energy deposition(Fig.4 (b)), the period of repeated movement became shorter, approached the period of laser repetitive pulses, while the moving distance of shock foot’s position was smaller. In the case of =15mm and =90 deg. model, without energy deposition(Fig.5 (a)) and with energy deposition(Fig.5 (b)), it is observed that flow in front of flare move on large scale and low frequency. Furthermore flow oscillation with energy deposition is larger than without energy deposition. It is not detected large scale oscillation in the case of =25mm and =90 deg. model with energy deposition(Fig.6 (a)) and without energy deposition(Fig.6 (b)).Fig.7 shows the drag vs. energy deposition in the case of =25mm and =30 deg. model. We can see that drag with energy deposition is higher than without energy deposition.
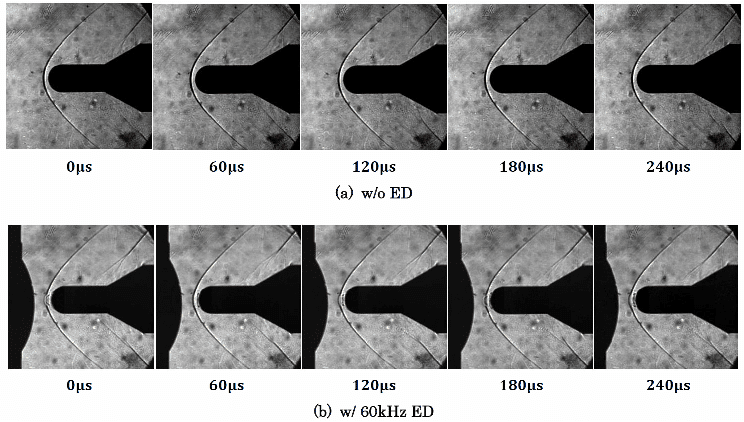
Fig.3 Schlieren images in l=15mm, θ=30deg. model
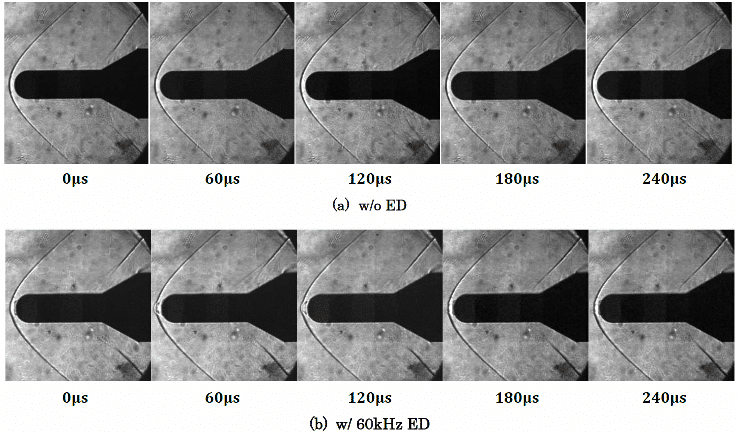
Fig.4 Schlieren images in l=25mm, θ=30deg. model
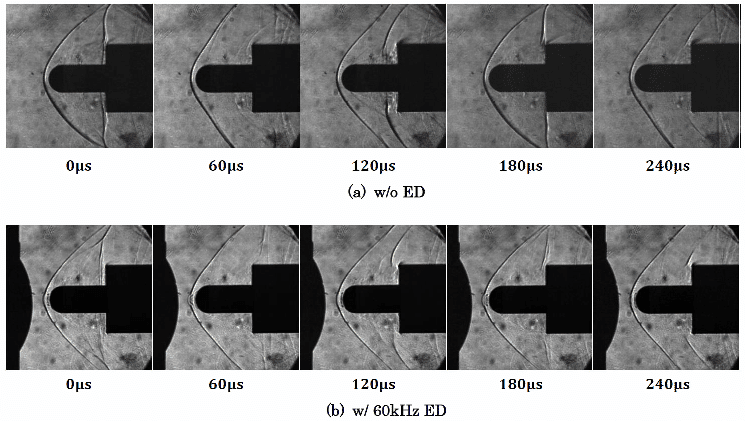
Fig.5 Schlieren images in l=15mm, θ=90deg. model
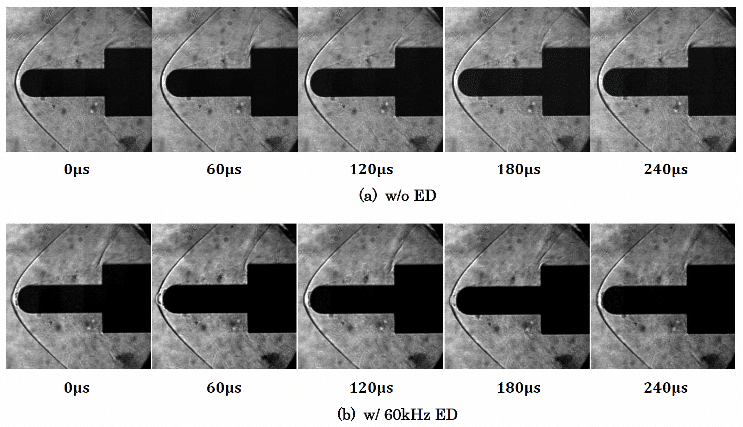
Fig.6 Schlieren images in l=25mm, θ=90deg. model
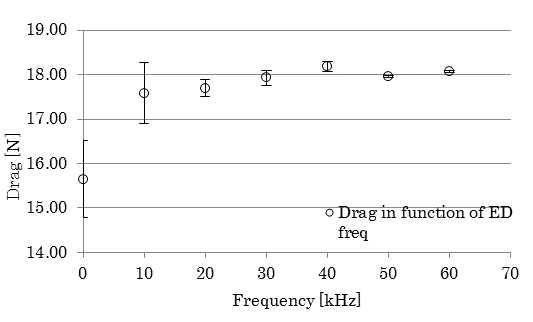
Fig.7 Drag in function of LED frequency in l=25mm, θ=90deg. model
4. Summary
The effects of repetitive laser energy deposition on the SWBLI over cylinder-flare models were investigated in a Mach 1.92 flow. The experimental results indicate that repetitive laser energy deposition can alter flow in the SWBLI region. It is observed that energy deposition delayed separation of boundary layer and suppress motion of shock foot in the =25mm and 30 deg. model. Also, in the =25mm and 90 deg. model, it is observed that energy deposition suppress oscillation of flow in front of flare.
Reference
[1] S. Raghunathan, “Passive control of shock-boundary layer interaction,” Prog. Aerospace Sci 25: 271-296, (1988)
[2] L. Bahi, J.M. Ross and H. T. Nagamatsu, “Passive shock wave/boundary layer control for transonic airfoil drag reduction,” AIAA 21st Aerospace science meeting, AIAA-83-0137:1-11 (1983)
[3] K. Kontis, N. Qin and J. L. Stollery, “Hypersonic performance of a lifting elliptic cone with and without strakes,” Journal of Spacecraft and Rockets 37 (1): 21-27, (2000)
[4]T. Osuka, E. Erdem, K. Kontis and A. Sasoh,”Laser Energy Deposition Effectiveness on Shock-Wave Boundary-Layer Interaction over Cylinder-Flare Combinations,” To be published in Physics of Fluid