In recent years, time-resolved particle image velocimetry (TR-PIV) has evolved as a means of measuring temporally correlated velocity fields, allowing the acquisition of PIV movies. Extension beyond roughly 10 kHz is not yet feasible with the diode-pumped solid-state lasers that typically are used in lower speed regimes. Instead, TR-PIV in high-speed flows is best accomplished using a pulse-burst laser, as this is the only light source capable of producing sufficient energy at the necessary pulse rates. Furthermore, it can produce pairs of pulses at arbitrary separation times, which allows the very short times between paired exposures compatible with high-speed flow, in contrast with the long time between exposures using a single kHz-rate laser. Simultaneous with the maturation of pulse-burst laser technology, quality high-speed cameras have begun to achieve desirable framing rates without excessive sacrifice of the size of the spatial array.
The present work represents the first use of pulse-burst PIV in a wind tunnel, and with few exceptions, the first pulse-burst PIV of any kind. The pulse-burst PIV system has been successfully operated in Sandia’s Trisonic Wind Tunnel (TWT), which permits experimentation up to Mach 3. Two initial applications are presented, one for a supersonic jet exhausting into a transonic crossflow and a second for transonic flow over a rectangular cavity. Further experiments should be available for the final paper, in which data will be acquired at higher Mach numbers to demonstrate capability for shock-driven flows. Together, these data sets establish the power of pulse-burst PIV to investigate the temporal development of turbulent structures in high-speed flow.
In the current work, a quasi-continuous burst-mode laser (QuasiModo-1000, Spectral Energies, LLC) with both diode- and flashlamp-pumped Nd:YAG amplifiers is used to produce a high energy pulse train at 532 nm. The pulse-burst laser generates up to 10.2 ms duration bursts every 8 seconds to a maximum repetition rate of 500 kHz. The maximum pulse energy is dependent upon the burst duration and repetition rate but greatly exceeds that possible with continuous-duty-cycle pulsed lasers. The laser is capable of producing doublets with variable interpulse spacing at all repetition rates, though in the present work the time between pulses in a doublet was 2.00 µs. In this work, 25 kHz and 50 kHz doublets were used for PIV measurements with energy per pulse of 50 mJ and 20 mJ for 10.2 ms burst duration and 175 mJ and 60 mJ for 2.2 ms burst duration, respectively. Images were acquired using two high-speed CMOS cameras (Photron SA-X2) which have a full framing rate of 12.5 kHz and an array of 1024 × 1024 pixels at this speed. Their windowing function allows the framing rate to be increased by sampling a semi-arbitrary portion of the imaging array. In the present case, each camera operated at 50 kHz with an array of 640 × 384 pixels. For the data included in this abstract, the two pulses in a doublet were frame-straddled around the cameras’ interframe transfer time, which allowed cross-correlation analysis of pairs of images; thus PIV velocity fields were acquired at 25 kHz. Additional data were acquired with the laser producing doublets of pulses at 50 kHz that were double-exposed in a single frame for auto-correlation image interrogation. The two cameras were placed side by side to extend the field of view in the streamwise direction to track the convection of turbulent eddies, yielding a combined field of view of approximately 70 × 21 mm2 for two-component PIV.
A velocity field sequence of the jet-in-crossflow experiment is shown in the attached figure. It was acquired at 25 kHz using cross-correlation analysis and is extracted from a much longer 10.2-ms burst, representing only an eight-snapshot, 280-µs portion of the full burst. Velocity fluctuations were found by calculating the mean velocity field over several wind tunnel runs and then subtracting it from each individual velocity field. The plots show in-plane velocity fluctuations superposed on the derived vorticity field. The sequence of velocity fields shows a counter-rotating pair of eddies entering the field of view on the left edge. As the pair convects downstream, the two eddies can be seen to rotate with respect to one another as they simultaneously drift further out in the y direction, reflecting the gradual trajectory of the jet. The clockwise rotating eddy (blue) appears to be ripping apart the counter-clockwise eddy (red), as its vorticity field becomes more fragmented with time. The stronger clockwise eddy begins to show this characteristic by the t=160 µs frame as well. As this eddy pair exits the downstream edge of the field of view, a collection of smaller vortical structures enters behind it. These are seen to spread out and weaken as they convect downstream. A global rotation of this collection of eddies is witnessed as well. By the final frame shown in the figure, the turbulent activity has become temporarily more quiescent.
The final paper will include data acquired from flow over a rectangular cavity at Mach 0.8, revealing the growth of shear layer structures and their recirculation within the cavity. The behavior of these large-scale structures in the shear layer is key to the acoustic tones produced by the cavity resonance and can be well explored using the temporally correlated velocity fields of pulse-burst PIV. Similar data also will be acquired prior to the conference at supersonic Mach numbers of Mach 1.5, 2.0, and 2.5, demonstrating the high velocities to which pulse-burst PIV may be applied.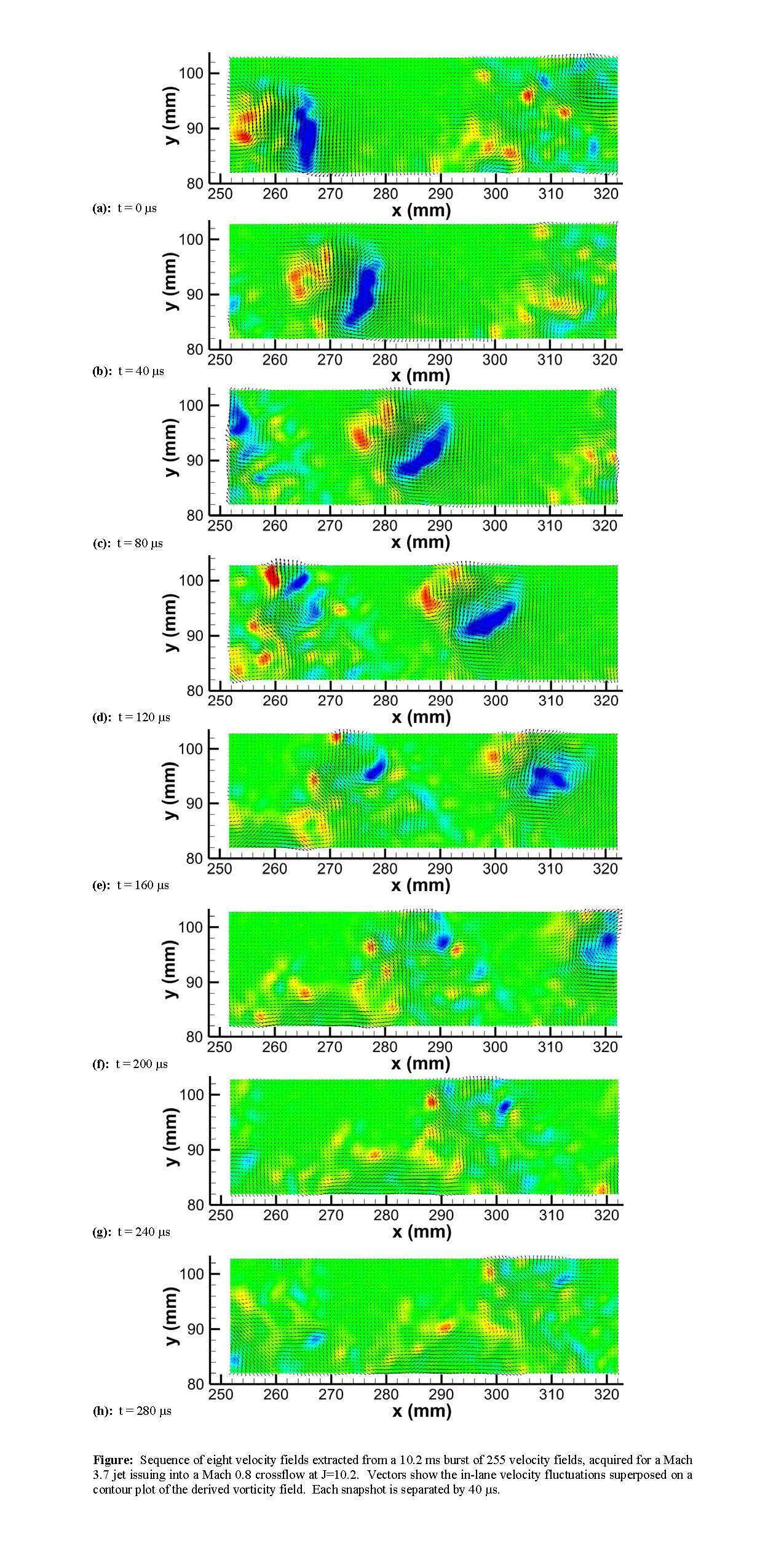