The numerical investigation of detonation propagation in a stoichiometrical hydrogen-air mixture flowing with supersonic velocity into a plane channel has been carried out with the purpose of determination of conditions that guarantee detonation stabilization in the flow without any energy input. The possibility of stabilization of formed detonation without energy input in the combustible gas mixture flowing with supersonic velocity into the plane channel with narrowing has been determined. For some Mach numbers of the incoming flow the method of determination of the channel shape which gives detonation initiation and its stabilization in the flow without any energy input is proposed.
Introduction
The interest in the study of detonation waves in a combustible gas mixture is closely connected with practical demands. So, the desire to use detonation in energy plants, for example, in detonation engines requires fundamental knowledge about the detonation process. In particular, the detail investigation of detonation propagation in a supersonic gas flow and determination of conditions that guarantee detonation stabilization are of a great interest. So, the method of detonation stabilization in a supersonic gas flow by means of weak additional discharges has been examined in [1].
In the present research the possibility of detonation stabilization without any energy input in the combustible gas mixture flowing with supersonic velocity into a plane channel was investigated.
Mathematical Model
Detonation propagation in a premixed stoichiometrical hydrogen-air mixture flowing into a plane channel (inflow cross-section and outflow one are perpendicular to the incoming flow direction) is studied. The combustible gas mixture under the normal conditions (p0=1 atm, T0=298K) is incoming into the channel with supersonic velocity that is essentially larger than the velocity of self-sustaining detonation propagation in the mixture at rest with incoming flow parameters: that is, M0>MJ0 (here, M0 is the flow Mach number, MJ0 is the Mach number of self-sustaining detonation).
A set of gas dynamics equations describing a plain, two-dimensional, nonstationary flow of the inviscid reactive multicomponent gas mixture coupled with detailed chemical kinetics equations [2] (in case of channels with narrowing) and [3] (for channels of special shape) has been solved using a finite-difference method based on the Godunov`s scheme. The size of mesh was selected so that the flow behind the detonation front (in particular, the flow in the induction zone) was represented correctly. Thus the computational mesh with cell size 0.03mm − 0.01mm was used in numerical calculations.
Numerical results
The possibility of stabilization of formed detonation without any energy input in the combustible gas mixture flowing with supersonic velocity into the symmetrical plane channel with narrowing was investigated. The initial instantaneous supercritical energy input in the narrow layer shaped domain was used for detonation initiation. Two detonation waves are formed as a result of the energy input: the first propagates downstream (this wave is transferred by flow) and the other one propagates upstream. The influence of geometrical parameters of the channel on propagation of the latter detonation wave has been studied. It has been established that for some values of the incoming flow Mach number M0 the geometrical channel parameters may be selected so that the detonation wave is stabilized in the flow. The pressure field and the density contours in case of detonation stabilization in the divergent part of the channel for M0=5.2 are presented in figure 1 (here y’=y/l, x’=x/l, where l is the half of the outflow section width; the arrow shows to flow direction). In this case the energy input in a narrow layer shaped domain containing the detonation front was used for wave stability testing. It has been established that the energy input (is equal 10J) with the exponential dependence of energy input density on transversal coordinate does not break the detonation stabilization.
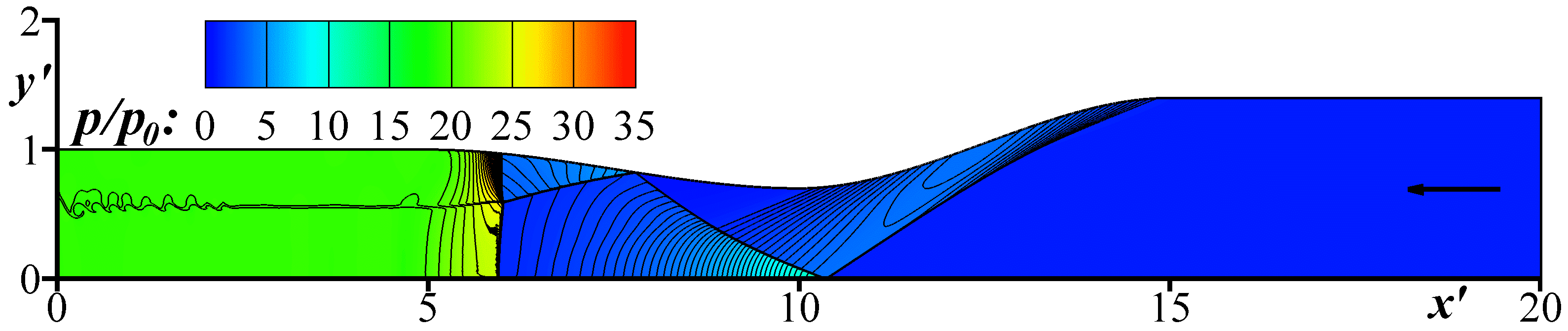
Figure 1.
For determination of channel shape which gives detonation initiation and its stabilization in the flow without energy input, a plane two-dimensional supersonic flow of the combustible gas mixture with Mach number M0 about the symmetrical semi-infinite plane obstacle placed along the stream was considered. The obstacle configuration was chosen so that the flow with detonation wave stabilized near the obstacle was formed. In case of M0=5.5 the structure of the stabilized ahead of the obstacle detonation wave was considered. It has been established that the detonation wave consists of three sections with different structures.
Then detonation combustion of the gas mixture flowing with the same velocity into plane channels (the top walls of which are determined by streamlines of the flow under consideration, the bottom one is determined by the plane of symmetry and by the obstacle surface) was studied. For some Mach number of the incoming flow, the possibility of formation of the stabilized detonation without any energy input in the channel, the shape of which is defined in this way, has been determined. The pressure fields in case of flow with the detonation wave stabilized ahead of the obstacle (a) and in case of formation of stabilized detonation in the channel of the special shape (b) for M0=5.5 are given in figure 2.
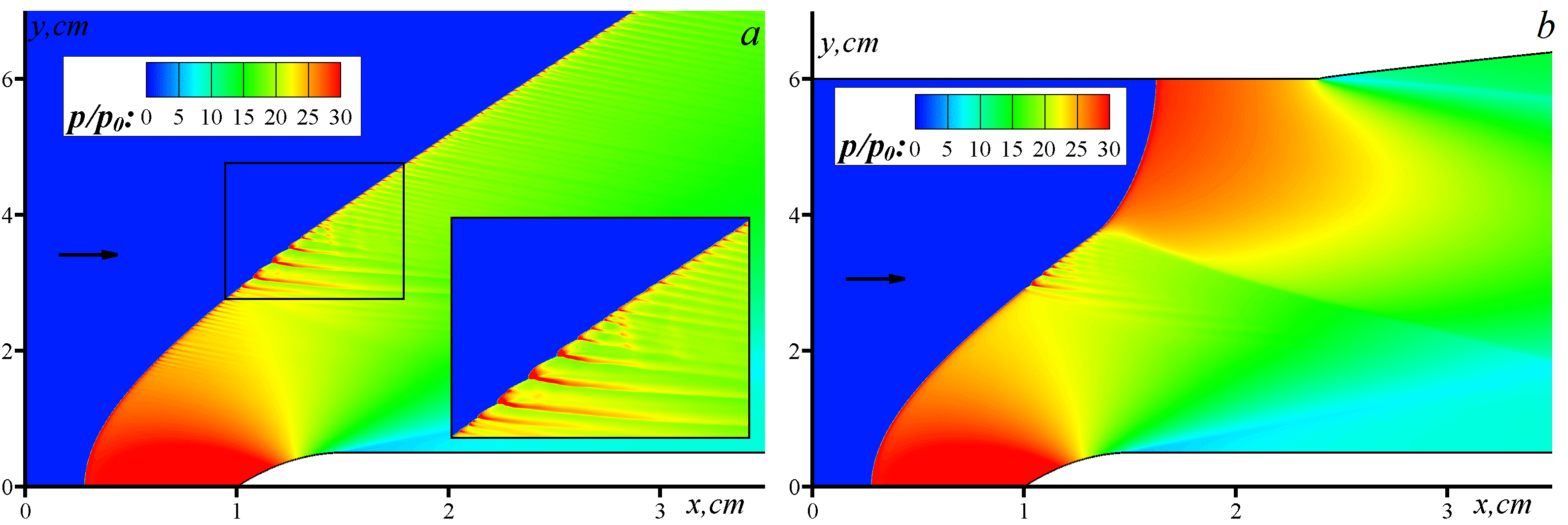
Figure 2.
Acknowledgments
This research has been supported by the Russian Foundation for Basic Research (14-01-00742a), by the President Grant for Support of Leading Science Schools of Russian Federation (NSh-5436.2014.1), by the Presidium of the RAS (special program) and by the Supercomputing Center of Lomonosov Moscow State University.
References
- 1. Zhuravskaya T.A., Levin V.A. 2012. Investigation of certain techniques for stabilizing detonation waves in a supersonic flow. Fluid Dynamics 47(6):793−801.
- 2. Warnatz J., Maas U., Dibble R.W. 2001. Combustion. Physical and Chemical Fundamentals, Modeling and Simulation, Experiments, Pollutant Formation. Berlin: Springer.
- 3. Starik A. M., Titova N. S., Sharipov A. S., Kozlov V. E. 2010. Syngas Oxidation Mechanism. Combustion, Explosion, and Shock Waves 46(5): 491-506.