The sonic boom estimation is necessary to develop supersonic transport vehicles. The typical sonic boom is produced by the shock waves generated from a supersonic transport vehicle cruising at more than the speed of sound. The shock waves pass through the real atmosphere and reach ground level. The sonic boom pressure signatures are affected by atmospheric conditions such as: turbulence, humidity, and temperature [1]. These atmospheric conditions cannot be controlled, however they must be considered. Atmospheric effects have to be well understood because it is extremely important to accurately estimate the sonic boom magnitude at ground level.
Turbulence effects are a critical issue for sonic boom estimation as sonic boom pressure signatures strongly vary by turbulence [2]. Lipkens et al. [3] found that in the case of shock-turbulence interaction, the averaged overpressure decreases, the averaged rise-time increases, and the standard deviations also increase. However, the turbulence effect is not yet fully understood because turbulence is by nature complicated; unsteady vortices, various length scales, and energy dissipated in a turbulence field are some parameters that add to its complexity. Since an effective means of creating turbulence in the laboratory are unsteady vortices, here, the effect of unsteady shock-vortex interaction is addressed.
Investigating the shock-vortex interaction is fundamental for producing a basic understanding of the shock-turbulence interaction phenomenon because a turbulent field consists of vortices. Two-dimensional numerical simulations of shock-vortex interaction have been conducted in previous studies [4, 5]. However, two-dimensional flow structure cannot simulate the real turbulent nature because the real turbulent field consists of three-dimensional tensile vortices. A vortex shape varies with time and moves randomly in turbulent field, resulting in relative velocity variations around the vortex.
To fully understand shock-turbulence interactions, investigating three-dimensional shock-vortex interactions is one of the solutions. Mariani et al. [6] experimentally investigated shock interaction with a uniform vortex loop shape in an open-ended shock tube facility. High-speed vortex loops are generally generated from the nozzle of the shock tube, and its shape is transformed depending on the nozzle geometry. A vortex loop’s shape, created from square and elliptic nozzles, varies with time [7], creating a naturally generated three-dimensional unsteady flow.
The objective of this study is an experimental investigation of unsteady three-dimensional shock-vortex interaction to understand the fundamental characteristics of shock-turbulence interaction. To generate a vortex loop, a shock wave generated by explosion energy of a flexible NONEL tube inserted into an aluminum hypodermic was propagated within a steel tube of 300 mm in length. The vortex loop was evolved from the square cross-sectional nozzle with side lengths of 22 mm. The vortex loop impinged on a reflected shock wave generated by the primary shock wave reflected from a wall located in front of the nozzle exit. The distance between the wall and the nozzle was 35 mm, 55 mm, and 75 mm. Advanced flow diagnostics: high-speed shadowgraph and schlieren photograph, and pressure measurements were used to provide insight into the flow physics.
The high-speed shadowgraph images of the unsteady three-dimensional shock-vortex interaction are shown in figure 1. The top and bottom rows denote Case 1 and 2; the wall distance is 35 mm and 55 mm from the nozzle, respectively. The distorted shock wave was transformed depending on the impinging vortex loop shape. The vortex loop shape was different between Case 1 and 2 before the shock impingement, namely the shape of the vortex loop varied with time. The vortex loop has an induced velocity around the vortex core, and the induce velocity vector is changed when the vortex loop is transmitted. In the present result, the vector might affect the shape of the distorted shock wave. To make the characteristics of the induced velocity due to the vortex loop obvious, the velocity distribution is measured using high-speed particle image velocimetry.
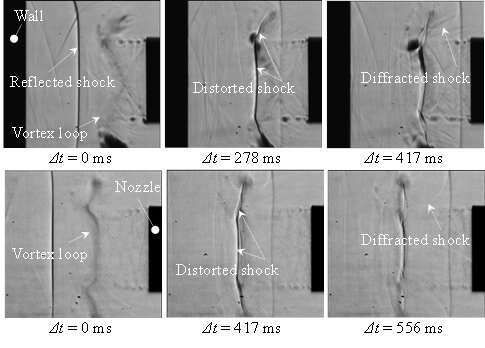
Figure 1. Continuous high-speed shadowgraph images. Top row: wall distance 35 mm; bottom row: wall distance 55 mm.
References
[1] J. Kane, “Some effect of the atmosphere on sonic boom,” Proceedings of Sonic Boom Research, NASA SP-147 (1967).
[2] A. Hilton, V. Huckel, D. J. Maglieri, “Sonic-boom measurements during bomber training operations in the Chicago area,” NASA Technical Note, NASA TN D-3655 (1966).
[3] Lipkens, D. T. Blackstock, “Model experiment to study sonic boom propagation through turbulence. Part I: General results,” J. Acoust. Soc. Am. 103 (1), pp. 148-158 (1998).
[4] L. Ellzey, R. M. Henneke, P. J. Michael, S. O. Elaine, “The interaction of a shock with a vortex: Shock distortion and the production of acoustic waves,” Physics of Fluids 7, 172, pp. 172-184 (1995).
[5] Zhang, Y. T. Zhang, C. W. Shu, “Multistage interaction of a shock wave and a strong vortex,” Physics of Fluids, 17, 116101 (2005) pp. 1-13
[6] Mariani, M. K. Quinn, K. Kontis, L. Marraffa, “Shock-free compressible vortex rings impinging on a stationary surface: Effects of surface angle variation,” Experimental Thermal and Fluid Science 47, pp. 126–142 (2013).
[7] Zare-Behtash, K. Kontis, N. Gongora-Orozco, K. Takayama, “Compressible vortex loops: Effect of nozzle geometry,” International Journal of Heat and Fluid Flow 30, pp. 561-576 (2009).