Introduction
The interactions between a shock wave and various flow fields are important research subjects in various fields. The interaction with energy deposited bubbles or turbulence are important issues in aerospace engineering. Energy deposition has been investigated in recent years as the method to deform a shock wave and reduce the wave drag of supersonic transport. Previous results indicated the drag was reduced up to 21% by the interaction with laser-induced bubbles at M=1.92[1], and suggested the hot elongated region like a dense bubble column is effective for drag reduction[2]. And the shock wave-a hot bubble column interaction was investigated using a single shock tube and a repetitive pulse laser, however the deformation of the shock wave was not observed since a strong shock wave could not be formed because of the limitation of the facilities[3]. Also, the interaction between a weak shock wave and turbulence has been investigated for the estimation of sonic boom propagating through the atmospheric turbulence. Some reports indicated that a modulation of a weak shock wave is greatly affected by turbulence, however the mechanism of the modulation has not been well understood yet.
Many shock wave interaction experiments have been conducted in a shock tube. In a previous study, the interaction between a reflected shock wave and turbulent flow induced in post shock flow behind an incident shock wave was investigated in a single-driver shock tube[4]. However, in a single-driver shock tube, the conditions of the reflected shock wave and the post shock flow are coupled with each other, cannot be controlled independently. If a shock tube has two driver sections, it can generate two shock waves and post-shock flows respectively and can control each condition independently. In this study, a Counter-Driver Shock Tube (CD-ST) has been developed, in which the interaction of the shock wave and the post shock flow can be investigated in various conditions. Using this the interactions between the shock wave and a column of bubbles generated by laser energy depositions or turbulent flow were experimentally studied.
Counter-Driver Shock Tube
Figure 1 shows the schematic of the CD-ST. The CD-ST has two Driver section (L-Driver and R-Driver) at the each end of the driven section (Fig. 1a). This configuration allows to generate Left- and Right-incident shock wave (L-iSW and R-iSW) independently, and Left- and Right-post shock flow (L-PSF and R-PSF) are induced behind each shock wave. R-Driver is activated with proper delay so that the interaction occurs in test section (Fig. 1b). After the head-on collision of the two incident shock waves, Left- and Right-transmitted shock wave (L-tSW and R-tSW) are formed as new shock waves. R-tSW is used as an interacted shock wave and is propagating to left while interacting with L-PSF in the test section (Fig. 1c). The condition of R-tSW and L-PSF are determined by the initial condition of each driver. Therefore the investigation of the interaction can be conducted on the CD-ST while the condition of the interacted shock wave and the flow field are controlled independently. Also, since the collision point of the shock waves was less than 34 mm in a demonstration, the CD-ST has an enough accuracy for the interaction experiments.
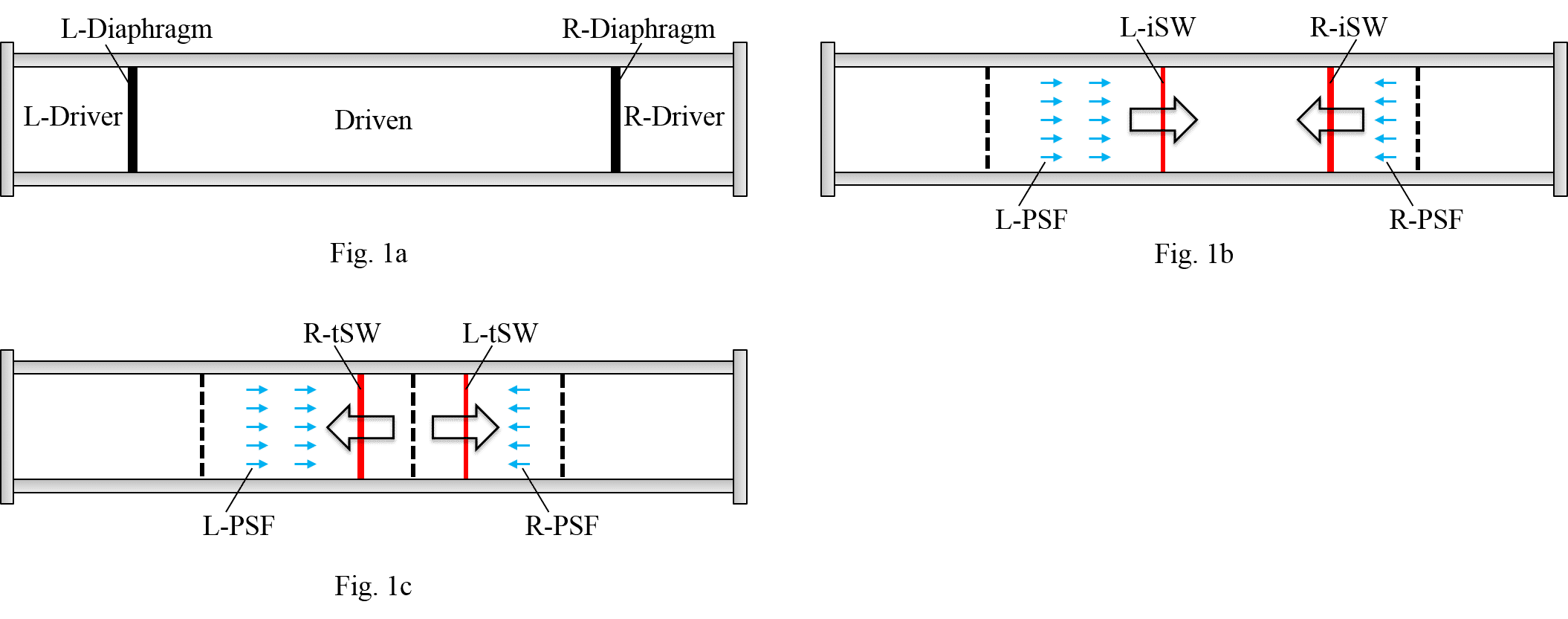
Fig. 1
Shock wave-a laser induced bubble column interaction
Figure 2 shows the experiment schematic of the shock wave-a laser induced bubble column interaction. The Mach number of L-iSW was set at low enough for the laser frequency so that a hot bubble column could be generated by a repetitive pulse laser. In L-PSW, a hot bubble was generated at a focal point after the previous bubble has been drifted by the flow, therefore a hot bubble column was formed in L-PSF (Fig. 2a). After the collision of the shock waves, R-tSW propagated to left and interacts with the bubble column (Fig. 2b). In the experiment, the Mach number of R-tSW was 1.27 and the velocity of L-PSF was 11.9 m/s.
Figure 3 shows the Schlieren images of the experiment. The distance between bubbles is calculated as 3.0 mm by the velocity of L-PSF and the frequency of repetitive pulse laser. Since the distance is calculated shorter than the diameter which is 1.8 mm from Schlieren images, the dense bubble column which the bubbles connected each other was generated. However, the deformation of the R-tSW has not been observed yet since the shock Mach number of the interacted shock wave is still too small.
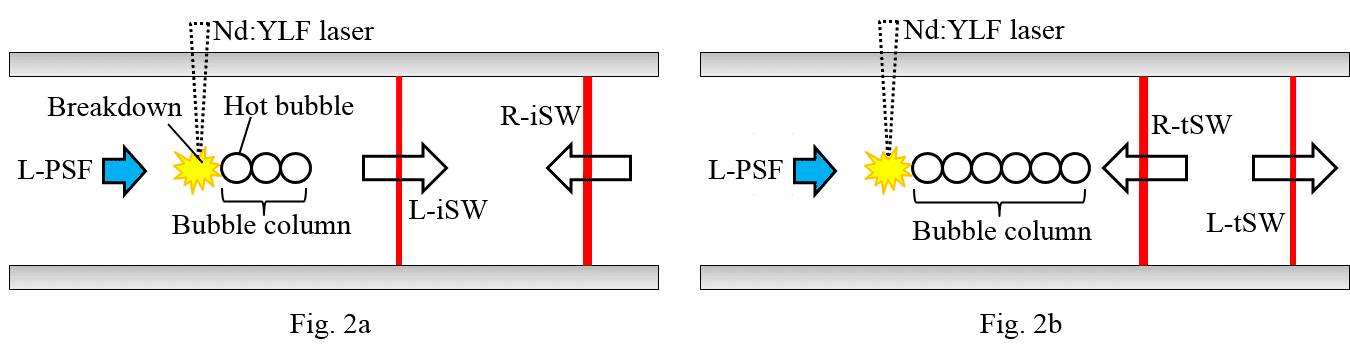
Fig. 2
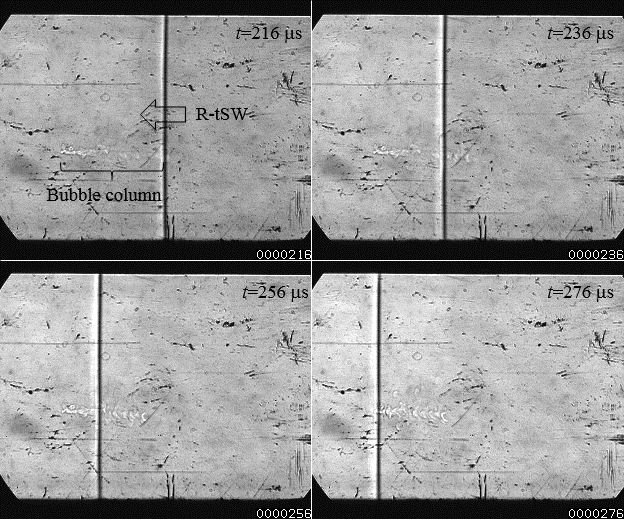
Fig. 3
Shock wave-grid turbulence interaction
Figure 4 shows the experiment schematic of the shock wave-grid turbulence interaction. In this experiment, the grid turbulence was generated by a grid in L-PSF (Fig.4a). After the collision of shock waves, R-tSW propagated to left and interacted with the grid turbulence (Fig. 4b). In the experiment, the Mach number of R-tSW was 1.14 and the standard deviation of the grid turbulence was 2.2 m/s.
Figure 5 shows the pressure history of the experiment at the different position of the CD-ST. The result suggests that the average pressure fluctuation did not change due to the interaction because of the weak interaction. More experiments need to be done in the CD-ST to obtain reproducible characteristics.
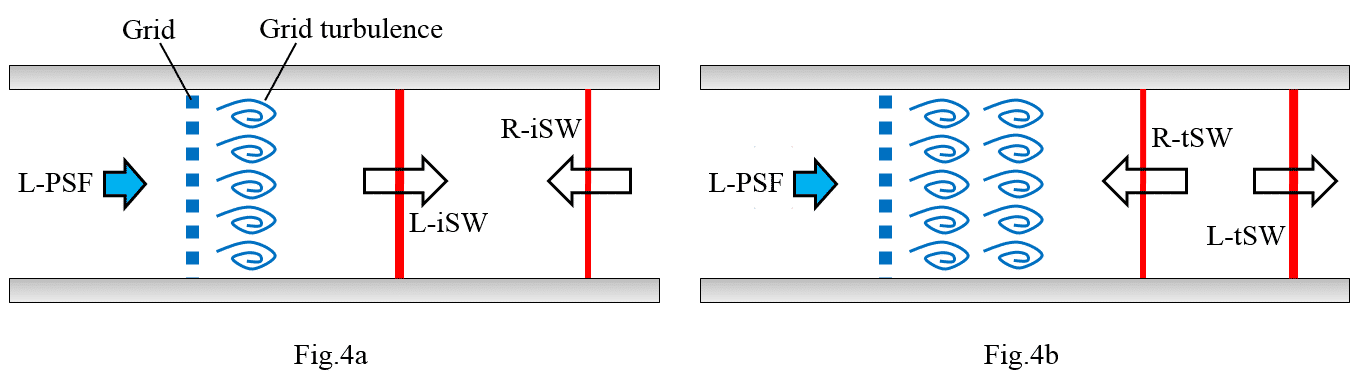
Fig. 4
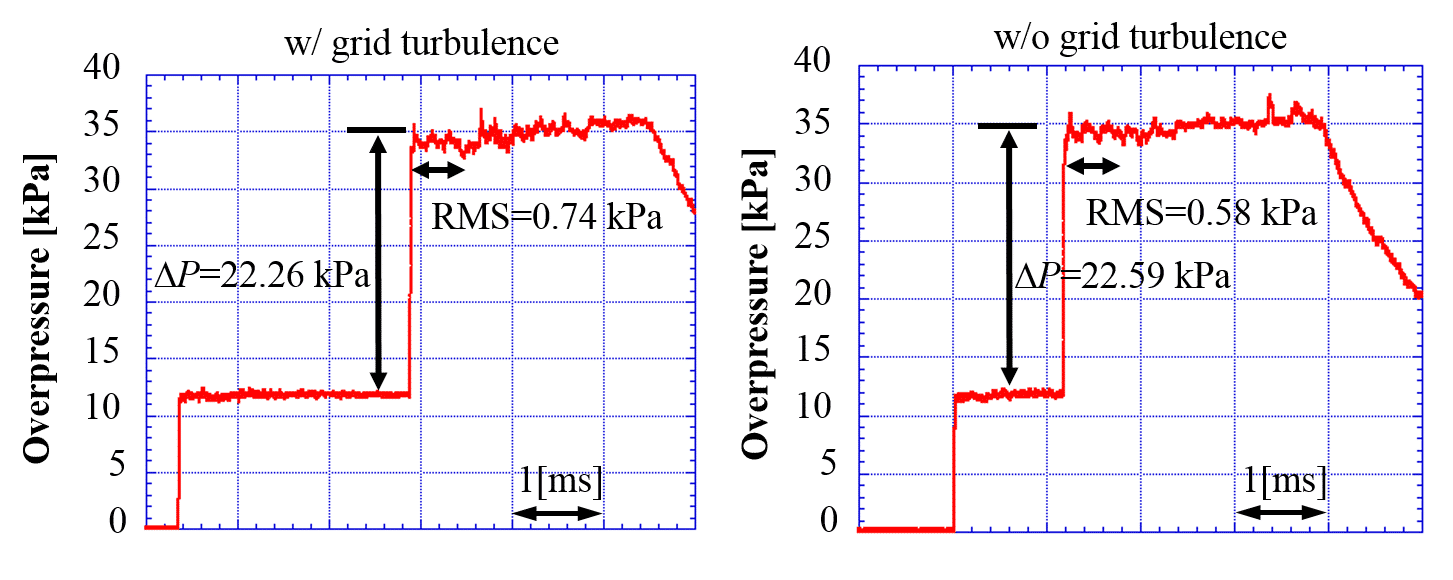
Fig. 5
Reference
[1]Kim, J. H., Matsuda, A., Sakai, T., and Sasoh, A., AIAA J. 49, 2076–2078 (2011).
[2]Kremeyer, K., Sebastian, K., and Shu, C. W., AIAA J. 44, 1720–1731 (2006).
[3]Takeya, K., Yokota, S., Sasoh, A., Proceedings of 44th Fluid Dynamics Conference, 1A12 (JSASS–2012–2015–F/A).
[4]Agui, J. H., Briassulis, G., and Andreopoulos, Y., J. Fluid Mech. 514, 143-195 (2006).