The FIRE II vehicle was flown in the 1960’s as part of the Apollo flight program with the aim of collecting extensive information on the heating environment experienced by a blunt spherical vehicle re-entering the terrestrial atmosphere from a lunar return velocity [1–3]. Flow conditions encountered by the FIRE II vehicle ranged from high altitude, high velocity, highly non-equilibrium flow, to near-equilibrium flow at low altitudes and velocities. Data collected from this vehicle remains some of the most comprehensive available on the shock layer heating environment experienced during Earth re-entry, and has been used extensively in the validation of numerical techniques [4–8]. The broad range of flow conditions experienced by the FIRE II was exploited in a recent experimental study by Capra and Morgan [9] aimed at measuring the heating environment on a 1:27.7 binary scaled test model. In this study, Capra and Morgan hypothesized that the total heat transfer expected on the binary scaled test model can be estimated from the measured flight values using Equation 1, for weakly coupled radiating flows where the heat loss from radiation does not significantly change the flow condition. Key to the formulation of this scaled heating is the invariance of radiative heat transfer between binary scaled models in a weakly coupled radiation flow, while the convective component scales with the binary scaling length scale, ls = Lflight / Lexperiment. Experimental results, shown in Figure 1(a), were shown to agree well with the assumption of invariant radiative heat transfer, agreeing to within 5 – 15% of the flight value.
qscaled = ls(qconvetion)flight + (qradiation)flight Equation 1
The present study aims at further investigating the proposed invariance of radiative heat transfer in a weakly coupled flow through computational analysis of full size, and appropriately sized binary scaled models and flowfields. The first stage of this study will focus on numerically re-creating the experimental conditions and results reported by Capra and Morgan [9], preliminary results of which are shown in Figure 1(b). The full size vehicle, at the appropriate flow conditions, will then be numerically reproduced. The second stage focuses on the numerical investigation of the vehicle (full size and scaled) over the peak heating environment when radiative heat transfer was more strongly coupled with the flowfield. For all models, the stagnation and surface heat transfer will be computed including the convective and radiative components. Total heat transfer will then be compared to empirical correlations as well as the proposed scaling given in Equation 1.
All numerical simulations will be performed with the compressible flow solver Eilmer, developed at the University of Queensland. The Eilmer flow solver discretises the compressible Navier-Stokes equations using a finite-volume technique. The solver has a number of upwind schemes available for the computation of the convective fluxes, while the viscous fluxes are computed using centred schemes. Eilmer includes physical models that can simulate the high-temperature gas effects in hypersonic flows. For this study, one- and two-temperature models are used to compute the shock layer flow over the FIRE II geometry. For flow conditions with weak coupling to the radiation field, the radiative heat transfer in the shock layer is computed as a post-processing step on the converged flow field calculation. Eilmer can couple to several radiation databases for that calculation of the radiation field. For more strongly coupled flows, we use a loosely-coupled technique to iterate on flow field and radiation field calculation. An assessment of the various modelling techniques is presented as part of this study.
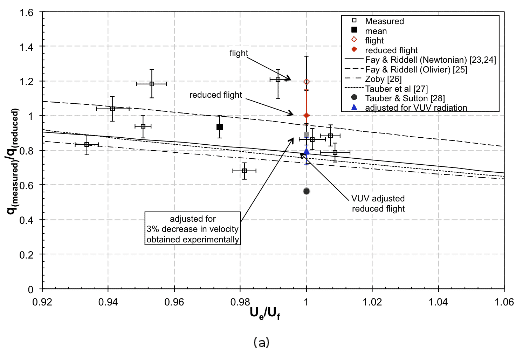
Figure 1: (a) Experimental results of heat transfer to a 1:27.7 binary scaled model of the FIRE II [9] and (b) Preliminary numerical results (temperature) of the flowfield around the 1:27.7 scale model.
References:
[1] Cornette, E. S, “Forebody Temperatures and Calorimeter Heating Rates Measured During Project Fire II Reentry at 11.35 Kilometers per Second” Technical Report, NASA-TM-X-1389, 1966
[2] Cauchon, D. L,“Radiative Heating Results from the Fire II Flight Experiment at a Reentry Velocity of 11.4 Kilometers per Second”, Technical Report, NASA-TM-X-1402, 1967
[3] Slocumb, T. H Jr,“Project Fire Flight II Afterbody Temperatures and Pressures at 11.35 Kilometres per Second (37 200 Feet per Second”,NASA TM X-1319, 1966
[4] Sutton, K, “Air Radiation Revisited” In Thermal Design of Aeroassisted Orbital Transfer Vehicles, Progress in Astronautics and Aeronautics, ed. HF Nelson, Vol.96, 1985, pp. 419-441, AIAA
[5] Balakrishan, A, Park, C and Green, M J, “Radiative Viscous Shock Layer Analysis of Fire, Apollo and PAET Flight Data”, in Progress in Astronautics and Aeronautics, Vol. 103, 1986, pp. 514-540
[6] Greendyke, R. B and Hartung, L. C, “Convective and Radiative Heat Transfer Analysis for the Fire II Forebody”, Journal of Spacecraft and Rockets, Vol. 31, No. 6, 1995, pp. 586-594
[7] Olynick, D. R, Henlin, W. D, Hartung, L. C and Candler, G V, “Comparison of Coupled Radiative Flow Solutions with Project Fire II Flight Data”, Journal of Thermophysics and Heat Transfer, Vol. 9, No. 4, 1995, pp. 586-594
[8] Wright, M, Loomis, M and Papapopoulos, P, “Aerothermal Analysis of the Project Fire II Afterbody Flow”, Journal of Thermophysics and Heat Transfer, Vol. 17, No. 2, 2003, pp.240-249
[9] Capra, B.R and Morgan, R.G "Total Heat Transfer Measurements on a Flight Investigation of Reentry Environment Model" Journal of Spacecraft and Rockets, 2013 Vol. 50 no.3 pp 494-503 (DOI 10.2514/1.A32333)