As an elementary process of high speed liquid jetting and atomization, the deformation and breakup of liquid drops in gas flow has been widely investigated. Recent researches conclude that Rayleigh-Tayler piercing and shear induced entrainment are the two primary mechanisms behind all known aero-breakup phenomena [1, 2]. The dynamic detail of the mechanisms and the presentation of varied minor effects are still open to question though. The current paper investigates the breakup of an isolated millimeter liquid drop subsequent to the interaction of a planar shock wave, with focus on the morphology of the early-stage deformation and the inception of interface instability.
The experiments are carried out in a square-cross-sectional shock tube with an electrical diaphragm rupture system. Direct high-speed photography is employed to record the interfacial flow. Fig.1 shows the sequential images of the deformation and breakup of a 2.5mm diameter water drop. The Mach number of incident shock wave is 1.39. The early-stage deformation is found to consist of simultaneous global flattening and regional erosions. On the windward surface, erosion of the interface starts from a circle about 60 degrees downstream the stagnation and quickly expands to the equator, whereas the stagnation region keeps firm and smooth till the final disintegration. On the leeward side, counter flow due to separation vortexes raises skirt-like water rings from the surface. The number and shape of the outreach rings seem to be affected by parameters other than the Weber number. These two types of surface erosion become the breach and main source of the mist.
The distance of the drop surface relative to the mass center is extracted via digital image processing, as shown in Fig.2. By this method, the scale of the smooth stagnation region and the characteristics of the unsteady erosion zones under varied test conditions are inspected. Combined with theoretical instability analysis and numerical simulation, the mechanisms of the unsteady surface erosion processes are further investigated.
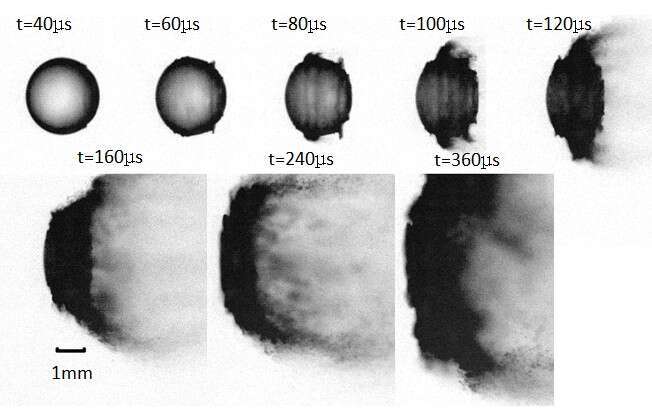
Fig.1 Sequential images of liquid drop breakup in shock induced flow (MS=1.39, drop diameter 2.7mm, We=3075, Oh=2.14×10-3, and Reg=6.4×104)
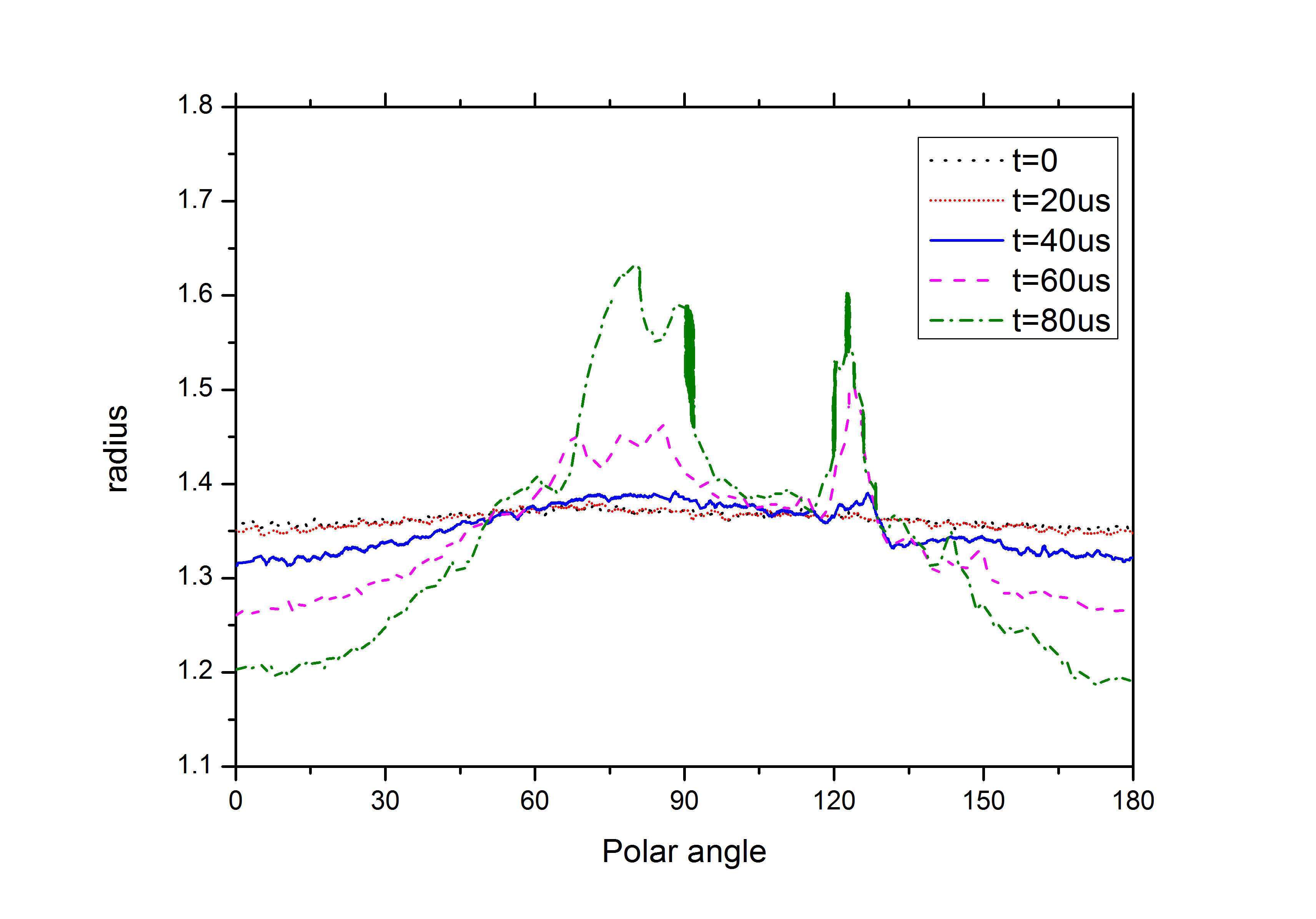
Fig.2 Distance of drop surface to mass center varying with polar angle at different moments corresponding to Fig.1.
References
- Theofanous, T. G. (2011). Aerobreakup of Newtonian and viscoelastic liquids. Annual Review of Fluid Mechanics, 43, 661-690.
- Joseph, D. D., Belanger, J., & Beavers, G. S. (1999). Breakup of a liquid drop suddenly exposed to a high-speed airstream. International Journal of Multiphase Flow, 25(6), 1263-1303.