ABSTRACT
The interaction of a shockwave with another shockwave is an unavoidable phenomenon in high speed flows. These interactions may lead to high pressure and thermal loads on the surface in the vicinity, deteriorates the aerodynamic performance of the system if present internally, and may also lead to the un-start of scramjet engine due to presence of the subsonic flow downstream of the interaction point. Edney (1) identified and classified these interactions into six different types using a blunt body in a Hypersonic Wind Tunnel. This shock-shock interaction phenomenon has attracted the interest of many research groups around the world especially in the hypersonic speed regime. Wieting et al (2) have experimentally studied the shockwave interference heating on a cylinder at Mach 6 and Mach 8. Sanderson et al (3) have also investigated the effect of shock-shock interaction in a dissociated high enthalpy flow.
Most important feature of these interactions is the increase in the pressure and heat flux on the nearby surface, where the waves after the interaction meet the surface. Though there has been some understanding of the fundamental flow physics associated with hypersonic shock interactions, the effect of energy content of the flow on the nature of these interactions and there different realms of occurrence are not clear. It is in this backdrop, the present study has been initiated in IISc to comprehensively map the surface convective heating as well as the static pressure on a hemispherical body placed in close proximity to shock-shock interaction zone at hypersonic Mach number.
For the present work, the Hypersonic Shock Tunnel–2 (HST-2), at Laboratory of Hypersonic and Shock wave Research (LHSR), Indian Institute of Science, is used to generate a flow of Mach 5.64, obtained by the straight through mode operation of the tunnel. Here, a wedge is used to generate a planar shock-wave, which is allowed to interact with a bow shock formed in front of a hemispherical body. The model assembly is designed in such a way that keeping the hemispherical body fixed, the wedge can be moved, thereby changing the distance between the wedge tip and nose of hemispherical body. Different location of the wedge relative to the hemispherical body leads to the change in location on bow shock where the planar shock interacts with it. Thus, by changing the relative location of the wedge with respect to the hemispherical body makes the interaction point to move along the trajectory of the bow shock from a low subsonic region of the bow shock to the near normal region of the bow shock near the nose region. This leads to the formation of different interaction patterns which were classified by Edney (1) as Type I to Type VI. For all the experiments reported here, air is used as the test gas at the following test conditions using HST-2. The typical experimental conditions are shown in Table 1.
TABLE 1. TYPICAL EXPERIMETNAL CONDITIONS ADOPTED IN THE PRESENT STUDY
Freestream Mach No.
|
Static Pressure (kPa)
|
Static Temperature (K)
|
Density (kg/m3)
|
Unit Reynolds No. (/m)
|
5.64
|
1.86
|
143.59
|
0.0508
|
6.98x106
|
The platinum thin film heat transfer gauges deposited on Macor substrate are mounted in flush along the centerline of the hemispherical body. The photograph of the model used in the present study is shown in Figure1.
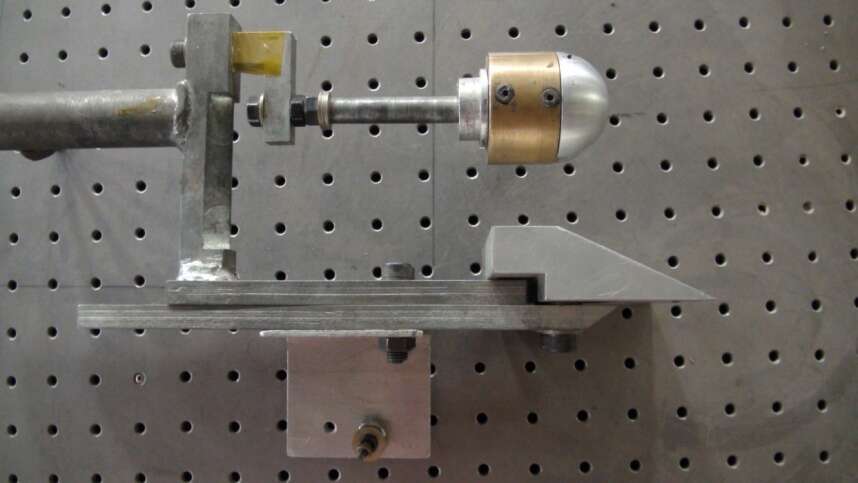
Figure1. – A photograph of the test model assembly used in the experiments
High speed flow visualization is carried out to understand and characterize different shock interaction patterns and the corresponding relation to the measured heat transfer rates and pressure distribution along the surface of the hemispherical body. The six different type of interaction patterns observed in the present study are shown in Figure 2.
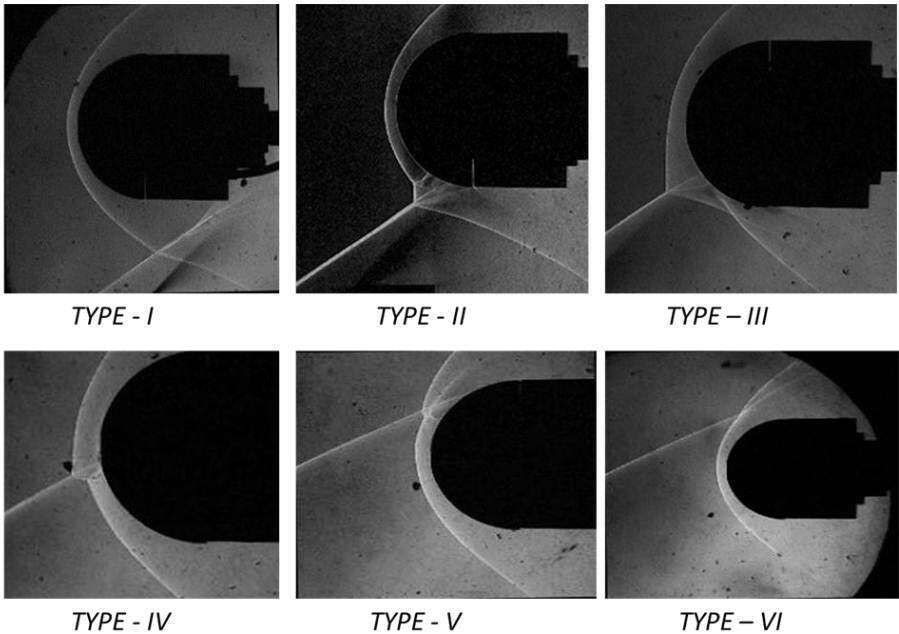
Figure2. – Schlieren images showing different shock interaction patterns.
Heat transfer and pressure measurement studies are currently underway and the details of the studies will be presented in the full length paper.
REFERENCES :
1) Edney. ‘Effects of Shock Impingement on Heat Transfer around Blunt Bodies’, AIAA JOURNAL, vol. 6, no. 1, January 1968.
2) Wieting, A.R. , Holden, M.S ‘Experimental shock-wave interference heating on a cylinder at Mach 6 and Mach 8’, AIAA JOURNAL, 27, 1557-1565 (1989).
3) Sanderson, S.R., Hornung, H.G., Sturtevant, B. ‘Aspects of planar, oblique and interacting shock waves in an ideal dissociating gas’, Phys. Fluids 15, 1638-1649, 2003.