Optimization of nozzle by increasing expansion ratios and reducing the size of divergent has becoming a real challenge due to the presence of flow separation and coupled complex shock structures inside the nozzle, which lead to significant mechanical and thermal loads. The knowledge of separation phenomenon in supersonic nozzle is important to predict the side loads and the stability of the exhaust plume. Both experimental and numerical studies have shown several shock patterns induced flow separation, which leads to specific pressure distribution along the nozzle structure and side-loads generation. The origin of flow separation in overexpanded supersonic nozzles can be investigated to define whether the phenomenon is due to the incoming boundary layer (upstream of the separation) or the excitation of the ambient pressure (downstream of the nozzle exit). The energy of the recirculation flow behind the Mach disk could lead to large unsteadiness of the shock at separation. Coupling this effect to the adverse gradient pressure along the nozzle wall and to the interaction of the shock with the boundary layer, lead to complex flow structures. A comprehensive review of nozzle flow separation is given by Hadjadj and Onofri [1]. Papamoschou and Johnson [2], among others, have investigated the stability of the separation phenomenon in experimental planar nozzle. Nevertheless, studies of the origin of shock-induced flow for canonical nozzle are not abundant in the literature. In the present work, Large Eddy Simulations (LES) are carried out to investigate the flow separation and the complex shock wave / boundary layer interactions associated with shock-induced unsteady flows in TOC, TIC and conical nozzles. High-order WENO scheme based 3D numerical flow solver equipped with immersed boundary method is used for this purpose. An LES wall-model, which account for Adverse Pressure Gradient (APG) is developed to overcome the low-resolution of the very thin incoming boundary layer. Different nozzle flow regimes are investigated by varying the Nozzle Pressure Ratio (NPR). The computational results are compared with the experimental data for validation. Modal analysis based on Proper Orthogonal Decomposition (POD) is performed to study the dynamic behaviour of the jet structure at the nozzle exit. It has been shown that the flapping of the jet associated with the nozzle-lip vortex highly influences the low-frequency flow oscillations. Further analysis of the complex flow evolution including interaction of the shock with the incoming boundary layer (either laminar or transitional) will be presented in details in the full-length paper.
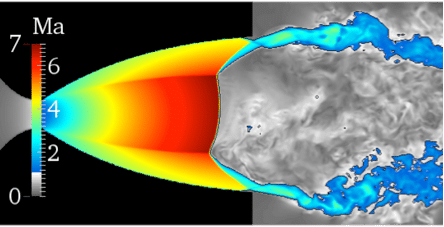
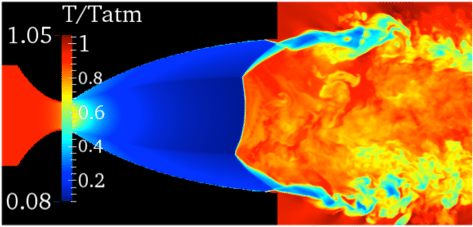