Particle analysis is a key discipline in safeguards (SG) and nuclear forensic (NF) investigations, as well as in environmental research. Particles-of-interest (POIs), such as “hot” (radioactive) particles, are usually in the micrometric size range, and intermixed within huge populations of other particles, like air-borne dust, soil, industrial exhaust pollutants or estuary sediments.
Characterization of individual POIs is accomplished by the Correlative Microscopy approach, which aims at providing complementary information of the same objects by using a variety of microanalytical tools. The first step in the analytical march in particle analysis consists of appropriate sample preparation methods. This step is followed by sequential application of a variety of microanalytical techniques. POIs must be identified, tagged, located, re-located, isolated, relocated and non-destructively analyzed. The final step in the chain (usually mass-spectrometry) may be destructive.
Uranium-containing POIs in environmental samples (ES) are of major interest for SG and NF. In November 2013 the first two authors launched a joint project aimed at particulate-level characterization of uranium-containing existing environmental reference materials (ERMs). A significant part of the project is to develop several innovative procedures, and to improve existing ones, for the toolbox available to the Particle Analyst. Our efforts have been concentrated in four areas:
- Sample preparation (an example is shown in Fig. 1):
- Dispersion of particles (in powder form) on stubs, planchets and thin-film tambourine-like substrates by the shock-wave dispersal device, developed at the IAEA.
- Fission Track (FT) detection and analysis:
- High resolution imaging of very large area “detectors” containing FT “stars” (Fig. 2).
- Automated segmentation algorithms for identification and location of FT “stars” in the “detectors” (a typical FT “star” is shown in Fig. 3).
- Laser Micro-Dissection (LMD) for retrieval (“harvesting”) of individual particles from the “catcher”, in which they are embedded (Fig. 4). The particles can then be lifted by micromanipulation and brought to further treatments and analyses.
- Exposing particles from their “catcher” shroud by plasma ashing to allow SEM examination.
- Alpha Track (aT) detection:
- Direct particle location using position-sensitive detectors (instrumental auto-radiography).
- Advanced analytical SEM (recently installed at the IAEA labs in Seibersdorf):
- Integration of TOF-SIMS with SEM search and EDS analysis (Fig. 5).
- FIBing individual particles to expose their inner structure (Fig. 5).
In this presentation, these procedures and preliminary results will be discussed.
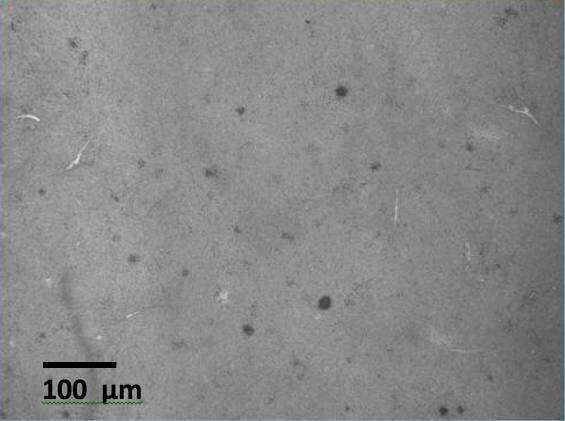
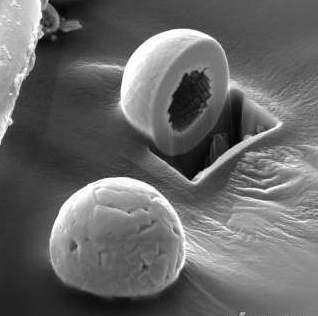
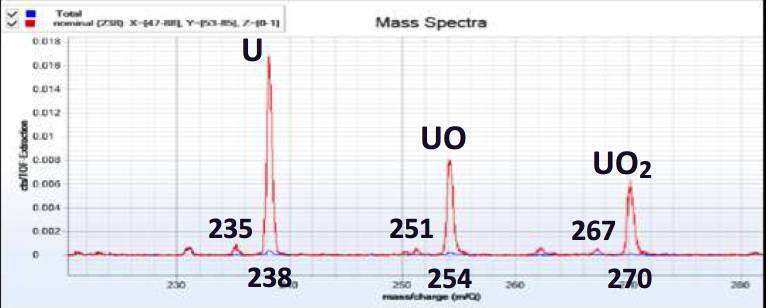
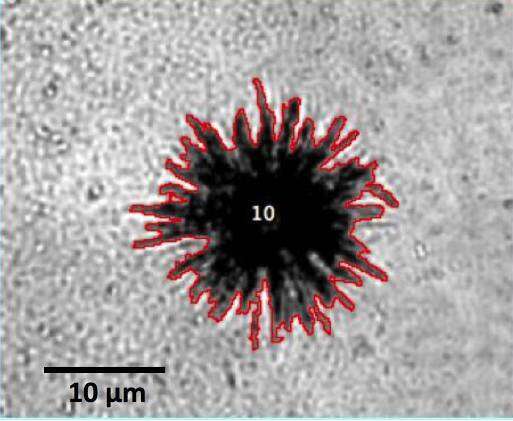
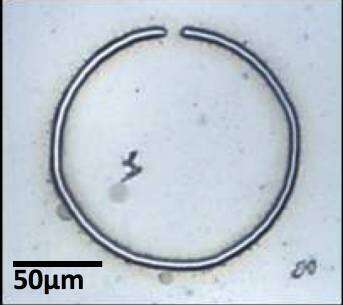
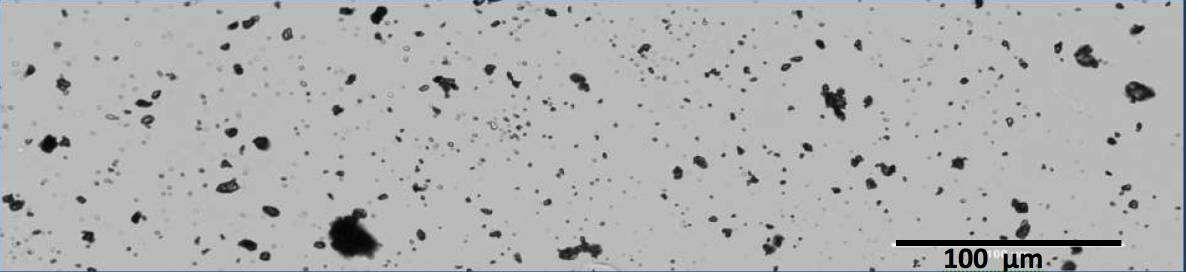